Academia.edu no longer supports Internet Explorer.
To browse Academia.edu and the wider internet faster and more securely, please take a few seconds to upgrade your browser .
Enter the email address you signed up with and we'll email you a reset link.
- We're Hiring!
- Help Center
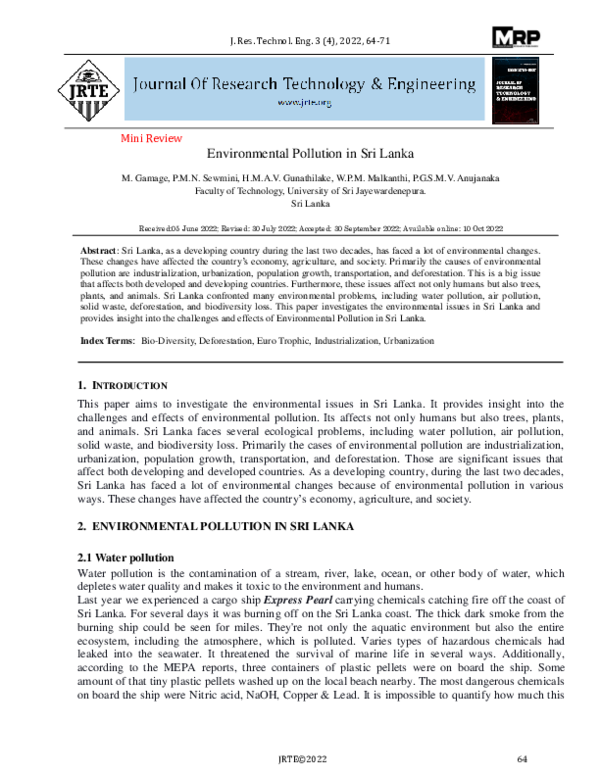
Download Free PDF

Environmental Pollution in Sri Lanka

Sri Lanka, as a developing country during the last two decades, has faced a lot of environmental changes. These changes have affected the country's economy, agriculture, and society. Primarily the causes of environmental pollution are industrialization, urbanization, population growth, transportation, and deforestation. This is a big issue that affects both developed and developing countries. Furthermore, these issues affect not only humans but also trees, plants, and animals. Sri Lanka confronted many environmental problems, including water pollution, air pollution, solid waste, deforestation, and biodiversity loss. This paper investigates the environmental issues in Sri Lanka and provides insight into the challenges and effects of Environmental Pollution in Sri Lanka.
Related papers
Environmental pollution is a severe hazardous status in Sri Lanka. It affects the atmosphere, land, and water in various situations due to the intervention of humans and nature. However, with the unlimited use of resources and harmful and unrestricted control of human beings, the effects are rising daily. Therefore, severe challenges are ahead for Sri Lankans due to environmental pollution. As a developing country, Sri Lanka is moving forward in the economy as an industrial sector, which causes to increase in energy consumption. There is a proportional relationship between energy consumption and wastage. If the percentage of energy consumption is high, wastage also rises. Sri Lanka faces many environmental issues, including inland, water, and air pollution. This report will explore those issues and their impacts on humans, animals, and the environment.
Sri Lanka is a tropical island that experiences beautiful environmental conditions and consists of many natural resources. Primarily Sri Lanka includes forests and biodiversity, minerals and water resources. The main objective is to provide a concise and up-to-date insight into the state of the environment and environmental challenges. Sri Lanka has suddenly acquired industrialization as a developing country, leading to increased energy consumption. When energy consumption rises, pollution to the environment also highly increases. At present, there are many environmental issues in Sri Lanka. But the most harmful and increasing problems are water pollution, air pollution and solid waste pollution. So, this article explores the environmental problems and their impacts on the people and animals and minimization of these issues in Sri Lanka.
Sri Lanka is presently faced with challenges of environment conservation such as deforestation, freshwater pollution, air pollution, noise pollution, soil erosion, wildlife poaching, coastal degradation, mangrove reduction, unsustainable development and bio piracy. Other problems are Land grabbing, water and jungle privatizations , piracy of indigenous knowledge etc. The aim of this research is to understand the environment issues in Sri Lanka and find out the solutions to protect nature. The researchers used the literature review as a secondary data collection method. Books, research papers and environment journals were used by researchers as a secondary data sources. Field visits and observations were conducted to collect primary data " s in the field. This analytical study concerned environmental degradation and conservation issues in Sri Lanka. Sri Lanka is one of the highest bio diversity hot pot in the world .The open economic policies and the implementations of unsustainable developments have been accelerated environment degradation. Government has an history of late implementation of rules and regulations such as Flora and fauna act, Costal conservation act, National environment act. Government and people have fundamental knowledge and they know how to protect nature. Nowadays, Earth needs democracy: it can conserve all biotic and abiotic factors on Earth.
Sri Lanka is an island on the southern tip of India, which has been identified as one of the top biodiversity areas in Asia region. Varied climatic conditions and topography of Sri Lanka has given rise to the unique biological diversity of the country. Sri Lanka, along with the Western Ghats of India has been classed as one of the 35 “Biodiversity Hotspots”. Sri Lanka is presently faced with challenges of environment issues such as deforestation, freshwater and air pollution, noise pollution, soil erosion, wildlife poaching, coastal degradation, mangrove reduction, climate change etc. Even though country is having several polices related to environment, yet environment pollution is continuing.
Sri Lanka is a tropical island in the Indian subcontinent which experiences wider array of environmental conditions and consists of many natural resources. The country primarily includes economically important agricultural land use systems, forests and biodiversity, minerals and water resources. The main objective of this chapter is to provide a concise and up to date insight to the state of the environment and environmental challenges, and governance in Sri Lanka. The first part of the chapter briefly describes the state of the natural resources in the country, the present trends and emerging environmental challenges under current development trajectories. Environmental pollution, land degradation and deforestation, degradation of biodiversity, negative impacts of climate change and waste disposal were identified as main environmental challenges. The second part highlights environmental impacts on agricultural, forestry, industrial and service sectors of the country. Final section summarizes the present state of the environmental governance and future prospects of the country under centralized as well as decentralized environmental management systems. Based on the information, policy suggestions and recommendations are also made. During past developmental era the resource base has been substantially diminished due to unsustainable use, but wise allocation and sustainable extraction can further improve the existing situation. Therefore, many initiatives from the government, private and other sectors have been taken for conservation and promotion of sustainable utilization. We recommend the accountability of the use of natural resources, effective way of low enforcement, enhance effectiveness of multi-disciplinary and multi-stakeholder consultation process to solve the environmental pollution, effective and compatible conservation of biodiversity outside protected areas and adopt an appropriate mix of centralized and decentralized mix of institutions for environmental governance in Sri Lanka.
Applied Economics & Business
Poverty, inequality, economic as well as social instability and environmental degradation are common features of unsustainable development. In general, the need for sustainable development is rarely at the top of priority lists of the people in developing countries because their livelihoods are mainly depended on available economic opportunities and environment variables. As a developing country, Sri Lanka has shown a progress of many aspects in sustainable development goals. However, irresponsible planning, unplanned development activities, absence of a proper monitoring as well as evaluation system and lack of knowledge on sustainable development has resulted in damaging the ecosystems over the last few decades. In this context, this study attempts to investigate the direction of some of the key environmental variables in the country. It also analyses the knowledge and attitudes of people in Sri Lanka towards the sustainable development, climate changes, forest as well as biodiver...
Man-made pollution and environmental degradation pose a severe challenge to all Sri Lankans. The main water resource problems in Sri Lanka are due to various human activities such as agriculture, fossil fuel combustion, urbanization, and industrial and commercial activities. Areas in every province where waste is not managed are causing a severe environmental problem due to the unnecessary pollution of its water by various pollutants.
International Journal of Environment, Engineering and Education
Water is indispensable for sustaining life, food production, economic growth, and well-being. However, the growing population and industrialization have intensified the demand for freshwater, posing significant challenges to water resources in Sri Lanka. This review paper focuses on understanding the types and causes of water pollution, with a particular emphasis on surface water pollution, as well as exploring preventive measures in the context of Sri Lanka. Given its severe consequences and the global issue of water scarcity, water pollution has gained attention from researchers, scientists, and organizations. Surface water bodies, such as lakes and rivers, face pollution primarily due to inadequate management of sewage and industrial effluents. Insufficient sanitation facilities in low-income settlements further exacerbate the problem, affecting the country. Despite existing regulations, the lack of monitoring allows improper waste disposal practices to persist. Rural areas exper...
Air pollution has become a crucial issue in the present world. The effects of Air pollution can be seen all over the world and it has been a hazardous matter since air is the most important factor for life to exist on earth. Air pollution usually happens when gaseous particles, biological molecules or other harmful substances are introduced in to air. There are many damages that happens to humans as well as animals that live on planet earth due to this Air Pollution. Food crops and cultivations can be affected by this as well. According to the 2012 world health organization's report about 7 million people have died as a result of air pollution.1 In most of the developing countries this problem of air pollution has been identified as a threat to the public life. Sri Lanka was a third world country and now in the present it has come to the level of " developing countries ". With this entry, more industries opened up and more number of vehicles were driven on the road. Accordingly the balance between the environment and the development was a little shaken. However the environmental pollution has increased at a bigger rate with the development of the country. Air pollution is the deadliest of all branches of environmental pollution. This paper would direct its readers to understand on what air pollution generally is, its status in Sri Lanka as the country we live in, its nature in the urban areas as compared to rural areas and the steps taken with regard to environmental law to either minimize the air pollution rates or to control it effectively.
Oralia, 2024
The Routledge Handbook of Cartographic Humanities,, 2024
Devonshire Archaeological Society Proceedings, 1995
Values, Objectivity, and Explanation in Historiography, 2017
Innovative Technologies and Scientific Solutions for Industries, 2020
International Journal of Multidisciplinary, 2023
Indonesian Journal of Community Engagement, 2016
Journal of paleolimnology, 2024
OncoImmunology, 2020
Educational Research for Policy and Practice
arXiv (Cornell University), 2020
Lecture Notes in Computer Science, 2006
Related topics
- We're Hiring!
- Help Center
- Find new research papers in:
- Health Sciences
- Earth Sciences
- Cognitive Science
- Mathematics
- Computer Science
- Academia ©2024
Advertisement
- Publications
This site uses cookies to enhance your user experience. By continuing to use this site you are agreeing to our COOKIE POLICY .
Grab your lab coat. Let's get started
Create an account below to get 6 c&en articles per month, receive newsletters and more - all free., it seems this is your first time logging in online. please enter the following information to continue., as an acs member you automatically get access to this site. all we need is few more details to create your reading experience., not you sign in with a different account..
Password and Confirm password must match.
If you have an ACS member number, please enter it here so we can link this account to your membership. (optional)
ACS values your privacy. By submitting your information, you are gaining access to C&EN and subscribing to our weekly newsletter. We use the information you provide to make your reading experience better, and we will never sell your data to third party members.
Already have an ACS ID? Log in here
Celebrate National Chemistry Week and get 30% off ACS membership with promo code NCW24 through Nov. 1.
Access more articles now. choose the acs option that’s right for you..
Already an ACS Member? Log in here
$0 Community Associate
ACS’s Basic Package keeps you connected with C&EN and ACS.
- Access to 6 digital C&EN articles per month on cen.acs.org
- Weekly delivery of the C&EN Essential newsletter
$80 Regular Members & Society Affiliates
ACS’s Standard Package lets you stay up to date with C&EN, stay active in ACS, and save.
- Access to 10 digital C&EN articles per month on cen.acs.org
- Weekly delivery of the digital C&EN Magazine
- Access to our Chemistry News by C&EN mobile app
$160 Regular Members & Society Affiliates $55 Graduate Students $25 Undergraduate Students
ACS’s Premium Package gives you full access to C&EN and everything the ACS Community has to offer.
- Unlimited access to C&EN’s daily news coverage on cen.acs.org
- Weekly delivery of the C&EN Magazine in print or digital format
- Significant discounts on registration for most ACS-sponsored meetings
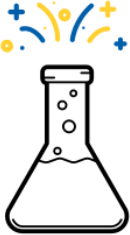
Your account has been created successfully, and a confirmation email is on the way.
Your username is now your ACS ID.
Grappling with the biggest marine plastic spill in history
The wreck of the x-press pearl unleashed a record 1,680 metric tons of plastic pellets on sri lanka’s coast in 2021. scientists want to understand more about the effects of this poorly studied type of marine pollution, by katherine bourzac, january 22, 2023 | a version of this story appeared in volume 101, issue 3.
Credit: Associated Press | A Sri Lanka navy soldier walks over mounds of debris from the X-Press Pearl on May 27, 2021.
- How can companies recycle wind turbine blades?
- Industrial ammonia production emits more CO 2 than any other chemical-making reaction. Chemists want to change that
- As nuclear waste piles up, scientists seek the best long-term storage solutions
- 10 years on, Flint still faces consequences from the water crisis
- Wind turbine blade recycling picks up speed
In 2021, a cargo ship caught fire and sank off the coast of Sri Lanka. The ship carried various chemicals, including 1,680 metric tons of plastic pellets called nurdles. Scientists are studying the accident, the largest known marine plastic fire and spill, to help advance our understanding of the environmental effects of this kind of pollution. Still, many unknowns remain about the ship’s cargo and its impact on the environment. The accident’s aftermath is playing out in Sri Lanka, where economic and political challenges present hurdles for local scientists and may complicate efforts to ensure compensation for environmental damages.
On May 20, 2021, a fire started on a cargo ship off the coast of Colombo, Sri Lanka. At home under COVID-19 lockdown, the country’s environmentalists and scientists watched media reports showing the ship spewing yellow and black fumes, and they worried that the X-Press Pearl would spill its oil into the sea.
But a few days after the ship caught fire, it became clear that the X-Press Pearl disaster was bigger than an oil spill. The ship was carrying more chemicals than its 348 metric tons (t) of bunker oil. Of the 1,486 containers on board, 81 held dangerous goods. The cargo included caustic soda, nitric acid, and fertilizer. The ship was also transporting polymers, including 1,680 t of plastic pellets, about 70 billion of them, each about 5 mm wide. These pellets, also called nurdles, are the raw materials that are melted and molded to make many plastic products.
On May 25, explosions were heard on the ship, and containers began falling into the ocean. Piles of plastic pellets meters deep engulfed the nearby Sarakkuwa beach. There was so much plastic that “you could not see the sand,” says Muditha Katuwawala, founder of the environmental organization the Pearl Protectors . “It was really scary.”
The accident is the worst ecological disaster Sri Lanka has ever faced and the worst marine polymer fire and spill in history. The largest previous pellet spill released 150 t into the waters of Hong Kong in 2012. In the wake of the X-Press Pearl accident, thousands of dead animals, including turtles, lionfish, and dolphins, were beached on the shores. Fishers lost their income, compounding the economic challenges of the pandemic. Meanwhile, the environmental and health risks posed by the chemical and polymer spill are still uncertain.
“This was an extreme incident, but it’s not an isolated one,” says Therese Karlsson , a science and technical adviser at the International Pollutants Elimination Network (IPEN).
Plastic pollution is ubiquitous. Nurdles and other plastic pollution can be found on just about every beach in the world. But little is understood about the chemistry, movement, and evolution of plastic pollution over time—crucial details for chemists who want to untangle its environmental impact. The X-Press Pearl spill offers a chance for chemists to learn more about this common contamination.
The Sri Lankan government has until June 2 of this year, 2 years after the ship sank, to file a claim in international court for damages against the ship’s insurance company. And the disaster is still unfolding, with consequences that may not be seen for years, scientists say. “There’s an acute phase and a chronic phase, the visible damage and the invisible,” says Meththika Vithanage , an environmental scientist at the University of Sri Jayewardenepura.
The incident and cleanup
The X-Press Pearl first reported problems on May 11, when the ship, operated by X-Press Feeders, was in Qatar’s waters. The crew reported to Hamad Port that a container was leaking nitric acid. The port denied them help off-loading the container, and the ship moved on to Hazira Port in Gujarat, India. On May 15, the Indian port also refused to help. According to a website that X-Press Feeders established to share incident information , both ports responded that “there were no specialist facilities or expertise immediately available to deal with the leaking unit.” The X-Press Pearl continued on.
On May 20, the X-Press Pearl , then anchored off Colombo, sent a Mayday call. Fumes were seen coming from the ship. A fire had started. The ship burned for days, releasing an estimated 8,000–13,000 t of air pollution . Firefighting ship crews from Sri Lanka and nearby India tried unsuccessfully to extinguish the fire with foams and water.
On June 2, the flames had died down enough that authorities tried to tow the boat farther away from shore, but it sank. By June 17, the ship had lodged on the seabed. It has remained there since, though workers began salvaging it in mid-November of last year and plan to recover the wreck in the first quarter of this year, according to the company’s website.
Ship fires used to originate mostly in the engine, but now many are chemical fires that start inside cargo containers, says Claudio Bozzi , a maritime law expert at Deakin University. And chemical fires on this scale are extremely challenging to put out, especially when it’s not clear what’s burning.
The risk of this type of fire is increasing as more ships sail with ever more cargo, Bozzi says. IPEN’s Karlsson notes that about 90% of the world’s goods are transported by sea. According to the insurer TT Club , ship fires occur about twice a month, and major fires happen once a month. “These ships are so large that any accident becomes catastrophic,” Bozzi says.
X-Press Feeder’s incident information website reports that “it is too early to tell how the fire started”; as of press time, the company’s public relations firm had not replied to a question about the cause of the fire.
The leaking nitric acid is a possible cause, Bozzi says. Nitric acid is a strong oxidizing agent that can ignite combustible materials, including wood and oil. He wonders whether the nitric acid container was stowed properly. To easily spot and address problems with a container of hazardous material, people should store it near a door or on the deck, not deep in the hold, he says. But port workers are not always aware of what hazards are on board and may not be properly trained on how to arrange cargo on these ships, he says.
The initial cleanup effort after the accident was massive, involving 18,973 people by June 14, 2021, according to a United Nations Environment Programme (UNEP) report . Once government efforts had cleared away much of the visible spilled plastic and other wreckage, such as twisted and burnt metal containers, the beach cleanup got a lot harder.
Over time, the plastic pieces started to blend into the environment and became harder to identify. Adding to the challenge was their varied color, shape, and size. Besides unburned nurdles, the debris includes a spectrum of burnt plastic, also called pyroplastic, that can be charred gray or orange or charcoal-like. Pyroplastics can also appear rocklike or resemble wood. Burnt plastic is brittle and readily fragments into smaller pieces. Some pyroplastic from the wreck is made up of nurdles that fused into larger pieces that look like seaweed or other organic matter.
The job of sifting through this hard-to-spot plastic has now mostly fallen to volunteers, the Pearl Protectors’ Katuwawala says. The Pearl Protectors has built handheld sieves for sifting the nurdles from the sand. Volunteers use them on cleanup days, and the Pearl Protectors leaves them on beaches for visitors to use on their own.
But the beaches are dynamic. One day a beach will be clean; the next, the movement of the tides and the waves might have brought up a batch of nurdles. Katuwawala says he has seen the plastic pollution move into the water and then get buried in sand during monsoon seasons, only to come back up later. “It’s not like you can clean one place and then it’s done,” he says.
Indian Ocean currents wrap around Sri Lanka. Six weeks after the spill, researchers documented severe nurdle pollution along 40% of the 340 km of coastline stretching from Kalpitiya to Matara in the south ( Mar. Pollut. Bull. 2022, DOI: 10.1016/j.marpolbul.2022.114145 ). Colombo is in the middle of that length of coastline. A citizen science mapping project called Nurdle Tracker Sri Lanka has recorded nurdles as far as the country’s east coast.
Whatever caused the fire on the X-Press Pearl , its aftermath spilled out into the waters around Sri Lanka and onto its beaches. Sri Lanka has so far received $7.85 million in compensation from the ship’s insurer. The government says this money has been used for cleanup efforts and to compensate fishers for lost income.
The spill disrupted fisheries around Sri Lanka. The University of Sri Jayewardenepura’s Vithanage says she saw “luminous green” water in the Negombo Lagoon, a major fishing area. Such green water is a sign of an algal bloom, something she had never previously observed in this region. Algal blooms can kill fish and contaminate them with toxic compounds. The bloom could have been caused by urea carried on the ship.
The accident occurred during the prime fishing season, says Hemantha Withanage, director of the Sri Lankan environmental advocacy group Center for Environmental Justice . The CEJ’s representatives interviewed local people, most of whom reported lost income after the accident. A joint report by the CEJ and IPEN recounts that some fishers found their nets damaged after the accident and observed nurdles in fish’s stomachs, gills, and mouths.
Other marine species were also affected by the spill. Most of the world’s sea turtle species visit the Sri Lankan coast at some point in their life cycle, and the disaster’s impact on turtles has been particularly stark—the X-Press Pearl was anchored in a turtle migration path. Over 600 turtles were found beached in the months after the disaster, some with broken shells and others with burns. Vithanage says that for every beached turtle, it’s likely another five turtles have died at sea.
The accident happened at the start of the monsoon season, when currents run to the north and pelagic fish, which normally live farther out to sea, enter coastal waters to spawn. This timing means there could be a drop in fish populations in coming years.
Wildlife was likely harmed by intense heat from the fire, explosions, and even sound waves. The UNEP report also points to chemical risks. There were 1,040 t of caustic soda and 25 t of nitric acid on board, both of which are water soluble. But both are much denser than seawater and may have sunk to form corrosive plumes on the seabed. These plumes could inflict chemical burns on wildlife such as turtles and dissolve animal carcasses. “We might not have seen some of the damage” for that reason, Vithanage says.
There are also questions about the exact contents of the ship and what happened to them during the fire. If the 210 t of methanol listed on the X-Press Pearl ’s manifest spilled instead of combusted, it would have floated on the water and created a cloud of toxic vapors that would have been harmful to fish and posed particular risks to turtles, dolphins, and other animals coming up for air, according to the UNEP report.
Another unknown is the 9,700 t of epoxy resin carried in 349 containers. The ship’s manifest does not indicate whether the resin was liquid or solid. Liquid epoxy could have formed a toxic plume on the seabed and traveled with the currents, burning swimming and stationary animals and plants, according to the UNEP report. UNEP recommends finding more information about this part of the cargo, such as the epoxy’s material safety data sheet, to evaluate the risks. As of press time, company representatives had not responded to questions about the epoxy resin.
Plastic pollution questions
While reactive chemicals from the spill may have caused acute environmental damage, environmentalists and researchers are also concerned about potential long-term issues caused by the X-Press Pearl ’s spilled plastics, which may persist in the environment for a long time.
Most news about plastic pollution focuses on end-of-life issues—for example, what happens after we throw away a plastic bottle or bag. But plastic can also leak into the environment earlier in its life cycle. About 230,000 t of nurdles is released into the environment every year. “Nurdles are one of the most well-recognized plastics in the ocean,” says Christopher Reddy , a marine chemist at the Woods Hole Oceanographic Institution (WHOI). “If you throw a net off a boat and trawl, you’ll get a nurdle.”
A handful of other large-scale nurdle spills are known, but they are orders of magnitude smaller than the 70 billion nurdles released by the X-Press Pearl . Several spills occurred in 2020 alone. That year, an estimated 731 million nurdles were spilled into the Mississippi River; an unknown tonnage was spilled off South Africa’s coast; and 13 t was spilled from a container ship in the North Sea. The scale of spills on the open ocean is more mysterious. Plastic is not considered a hazardous material, and international law does not require shipping companies to report all containers lost at sea.
The X-Press Pearl disaster gives scientists a unique opportunity to address some of the biggest questions about plastic pollution: How does it break down over time, what kinds of chemicals does it leach in the process, and how do those chemicals affect the environment? In the case of a spill like in Sri Lanka, “you have that provenance of where and when plastic entered the environment,” WHOI materials scientist Bryan James says. “You can do forensics, track it. You can estimate the baseline of what it is and how it changes over time.”
One particular issue scientists want to address with the Sri Lanka spill is what happens when plastic gets burned.
As the ship burned, some nurdles were released in relatively pristine form, maintaining their white color; others hit the ocean seared orange, flecked with gray ash, or fused to form rock- or seaweed-like black shapes. Chemists want to know how these changes could affect what molecules the nurdles release into the environment. Potential toxic compounds might include ingredients in the pellets themselves, molecules absorbed from other spilled cargo, or chemicals created during the fire.
Environmental scientist Vithanage has been documenting changes in nurdles washed up on Sarakkuwa beach near the shipwreck. Before the accident, she had sampled sand and nurdles (at the time quite scarce) from the beach. She’s been regularly sampling there since a few days after the accident, gathering nurdles from the surface of the sand and digging for them a meter deep. She’s seen the pellets wear down and shrink over time. She’s observed the nurdles get buried in the sand and come back up. Her ability to assay organic chemicals in and on the nurdles is limited by the equipment in her lab, she says. But she’s been testing them for metals.
Vithanage tested for lithium after learning that the ship’s cargo included a container of batteries. Her group found high levels of lithium in the water and on the nurdles ( Sci. Total Environ. 2022, DOI: 10.1016/j.scitotenv.2022.154374 ; Mar. Pollut. Bull. 2022, DOI: 10.1016/j.marpolbul.2022.114074 ). Her team also found high levels of chromium, which Vithanage believes may have come from coatings on the shipping containers. Exposure to chromium can slow plant growth, damage tissues in aquatic animals, and cause organ failure in people.
According to the cargo list, most of the nurdles on board were made of polyethylene, but that tells chemists little about their composition and what additives might be present. The CEJ collaborated with IPEN to test for toxic organic compounds in the recovered nurdles. The team found ultraviolet sensitizers, bisphenol A, and polycyclic aromatic hydrocarbons (PAHs), which are carcinogenic.
The PAHs either are from the ship’s fuel or are a by-product of plastic combustion, Reddy says. WHOI data in a paper currently under review show that some of the spilled nurdles have higher levels of PAHs than have ever been reported for ocean plastic. Reddy says it’s not clear whether these chemicals would be bioavailable. There has been very little research on how PAHs interact with pyroplastics. These carcinogens might cling tightly to the plastic’s surface, or they might shed into the sea or onto people’s skin.
Another reason that chemists are studying pyroplastics is that when plastic is burned, the chemistry gets more complex. Purdue University environmental engineer Andrew Whelton has studied this complexity in the context of plastic water pipes. In 2018, a wildfire in Santa Rosa, California, led to benzene contamination in the city’s water supply. Benzene was created when the plastic pipes burned, and the chemical persisted in the water system. Acute exposure to benzene can depress the central nervous system; chronic exposure can be carcinogenic and cause blood problems such as anemia. Organic molecules like benzene cling to plastic, an effect that’s more pronounced in salty water.
Without knowing the chemical details of the nurdles and other plastics carried on the X-Press Pearl , scientists can’t say what might have been released into the ocean. As a benchmark, Whelton says, consider that for every gram of high-density polyethylene that is burned, 0.8 µg of benzene gets released.
Whelton says more research is needed on how the plastics were transformed by the fire and what materials were released into the water. But this information is hard to come by.
Sri Lanka’s Marine Environment Protection Authority (MEPA) has convened a scientific committee to gather information about the accident’s environmental impacts. The committee submitted its report to the government in January as this article was going to press. C&EN contacted MEPA and members of the scientific committee with questions about what kinds of samples were gathered, what testing was being done, and what data they had collected on marine animal mortality. Committee members were reluctant to talk given MEPA’s stipulation that all findings must be kept secret ahead of potential international court filings.
Reddy and colleagues at WHOI are extensively testing a small volume of samples shared with them by Asha de Vos, executive director of Oceanswell , a nonprofit conservation research and education organization in Sri Lanka. When the ship caught fire, she contacted Reddy because he had experience working on oil spills, including the Deepwater Horizon disaster . She shared samples taken from Pamunugama, a beach just south of Sarakkuwa, 5 days after the fire started.
Those samples and others now sit in WHOI’s labs. At WHOI, James dons gloves and pulls plastic bags and glass jars full of debris from a refrigerator. One piece of agglomerated and incinerated plastic looks like a stack of toasted nori. Some of the burnt plastic bits look like fragments of wood or stones. The WHOI scientists have jars of white, orange, and gray nurdles sorted for study—they want to know how the degree of burning affects their chemistry and toxicity.
When probed with high-resolution mass spectrometry, most of the clean, white nurdles from the beach look similar—like high-density polyethylene ( ACS Environ. Au 2022, DOI: 10.1021/acsenvironau.1c00031 ). The burnt plastics are a widely varied group, with no nurdle an exact chemical match for its brethren. The WHOI group found long-chain hydrocarbons normally seen in petroleum, a mix of incomplete combustion products, degradation products of antioxidants, and more.
James and WHOI toxicologist Mark Hahn have studied the pellets’ potential for acute toxicity during zebrafish development, a standard animal model to test toxicity. They placed pellets of different colors—indicating different levels of burning—in the water with zebrafish embryos. They didn’t see acute toxicity in these studies.
The team then looked in more detail, assaying how exposure to chemicals leached from the plastic pollution affects human cells. They used solvents to coax relatively high levels of organic chemicals out of the different types of plastic pollution and then assayed whether they activated gene-expression-regulating proteins called transcription factors in the cells. Their goal was to identify biological pathways that might be activated by exposure to the plastic and assess whether burnt and unburned plastic from the spill had different effects.
The results are preliminary, but James says the team observed the activation of metabolic and endocrine pathways, as well as one associated with oxidative stress. Compared with material leached from pristine pellets, the burnt-nurdle cocktail had much stronger and more wide-ranging effects on cells. James says it’s not clear yet what this all means, but the WHOI team is expanding the tests to a cell model of marine life.
This kind of solvent-based leaching is not intended as a direct mimic of what happens to plastic pollution in the natural environment, Hahn says. It’s a kind of sped-up, worst-case scenario. “We’re getting things out of the nurdles that wouldn’t necessarily come out naturally,” he says.
The complexity of the plastics spilled from the X-Press Pearl is already overwhelming, Hahn says. “When you add on top of that combustion, photodegradation—it’s a wicked problem that’s not going to be easy to solve,” he says.
The hurdles
Scientists in Sri Lanka face not only this wicked problem but also logistic challenges. For example, there aren’t any facilities in the country capable of doing the kind of chemical analysis needed to detect trace levels of many kinds of harmful chemicals in beached fish. The country does not have extensive infrastructure for collecting environmental data or the necessary lab equipment to analyze them. And the country is facing an economic and political crisis, which means dealing with the environmental disaster is not a high priority.
Protesters frustrated with the country’s economic situation forced Sri Lanka’s president to step down in July. The cost of food, fuel, and other necessities has continued to rise. As of September, inflation in the country reached a record 69.8%. As a result, costs for scientists traveling from study site to lab to home are high.
Vithanage notes that all debris from the accident, including nurdles, is considered evidence for court proceedings. It must be turned over to MEPA for storage in a central facility that is managed by a different agency. Getting permission to do research on the nurdles is time consuming because of bureaucracy and the need to coordinate between multiple government offices, she says.
On Jan. 11, Sri Lanka’s Daily News reported that MEPA had turned over its report on the damages caused by the X-Press Pearl disaster to two other government officials. The paper reported that Urban Development and Housing State Minister Arundika Fernando said the country is “waiting to receive compensation amounting to US$ 6.5 billion for the damage caused to the coastline and coastal environment of this country due to this accident.” But the government must do more than wait—Sri Lanka has until June 2, 2 years after the X-Press Pearl sank, to file for damages in Singapore’s courts. In the same article, Fernando stated that they plan to do so. As of press time, MEPA chair Dharshani Lahandapura had not responded to C&EN’s query about the status of the report and its contents.
“There’s no compensation scheme in place for an accident like this, no mechanism to guarantee restitution,” IPEN’s Karlsson says. “These accidents have massive impacts on coastal communities, and they have to bear the brunt of the cleanup and the consequences.”
Bozzi, the maritime law expert, says there’s no question that the X-Press Pearl ’s owner carries liability for the spill and associated damages. “The burden of proof will be identifying and calculating the damages that need to be paid,” he says.
International shipping regulations ensure compensation for oil spills. When oil is spilled, “you don’t have to prove it would cause environmental damage; it’s just assumed that it does,” Bozzi says. That’s not the case for other kinds of spills, he says, “even though other forms of pollution are becoming more common.” He says many maritime regulations were written before microplastic pollution was even recognized, so these rules need to be revised.
Karlsson also hopes that the world can learn from the X-Press Pearl disaster and that international shipping laws can be updated before the next time something like this happens. For example, the International Maritime Organization’s Hazardous and Noxious Substances (HNS) Convention would ensure liability and compensation for damages from hazardous materials other than oil. But the conditions needed for the convention to take effect—including ratification by 12 countries—have not been met. Only 8 countries have signed the protocol. The HNS Convention would not cover plastic, but it does include other chemicals that were on board the X-Press Pearl .
And the next spill may be sooner than later. “With the increasing size of ships, increasing complexity of cargo, there will continue to be spills and accidents and continued risks to human health and the environment,” Karlsson says.
Meanwhile, chemists are digging in for years of research on the spilled nurdles’ environmental impact. Reddy, Vithanage, and other chemists hope research on the disaster can help advance scientific understanding of the environmental effects of plastic pellet pollution and serve as a case study for how to handle such spills in the future.
You might also like...
Sign up for C&EN's must-read weekly newsletter
Contact us to opt out anytime
- Share on Facebook
- Share on Linkedin
- Share on Reddit
This article has been sent to the following recipient:
Join the conversation
Contact the reporter
Submit a Letter to the Editor for publication
Engage with us on Twitter
The power is now in your (nitrile gloved) hands
Sign up for a free account to get more articles. or choose the acs option that’s right for you..
Already have an ACS ID? Log in
Create a free account To read 6 articles each month from
Join acs to get even more access to.
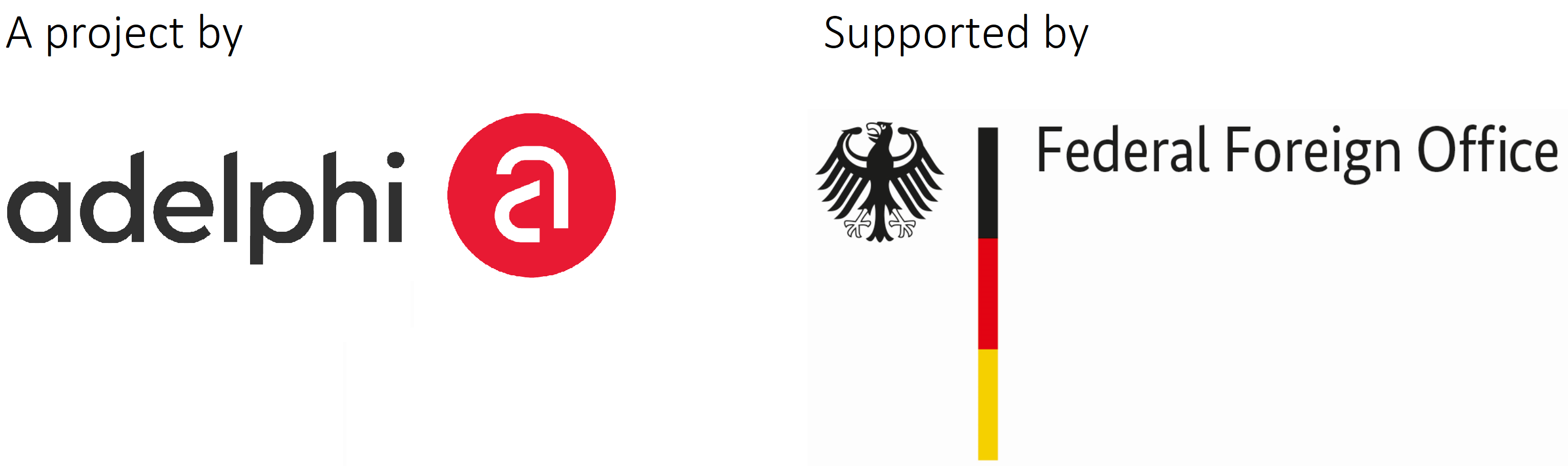
Main page content
- Environment
- Cooperation
- Development and Finance
Unravelling the environmental dimensions of the Sri Lankan crisis
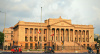
This article examines the environmental dimensions of the current crisis in Sri Lanka, the risks, and the way forward.
Sri Lanka, officially declared ‘ bankrupt ’ by its government, is facing the worst crisis in decades. Protesters stormed the Presidential palace in July 2022; and former President Gotabaya Rajapaksa fled the country. As protests against the newly formed government continue, political and economic uncertainties remain. While the crisis is mostly a creation of years of economic mismanagement by the Sri Lankan government, environmental concerns have also contributed to peoples’ hardship in the country.
Sri Lanka is extremely vulnerable to climate and environmental change. It is an island country that has not only lost a vast proportion of its biodiversity to developmental activities, but also is increasingly being battered by “ droughts, floods, landslides, cyclones, and coastal erosion .” Unplanned implementation of organic farming, unsustainable Chinese investments, the price spike caused by the Ukraine war, and a shipping disaster that continues to pollute coastal areas have structurally weakened living conditions on the island. Sustainable food production practices, renewable energy infrastructure, and climate adaptation efforts among others, could help to provide better solutions that bring stability and prosperity in the crisis-affected country.
An ‘Organic’ Debacle
As a part of its efforts to reduce dependence on imported fertilizers, and introduce eco-friendly measures with health benefits, the Sri Lankan Government under former President Gotabaya Rajapaksa declared a bold policy in 2019, switching to organic farming over a period of 10 years and imposing a ban on chemical fertilizers. While the policy is indeed forward-looking in an age of ecological crisis, which reinforces the need for food systems transformation, it was rolled out without any strategy and/or planning. In particular, the farmers were not equipped to switch to organic farming so quickly.
This move led to disastrous consequences for the vast majority of farmers , most of them being smallholders, whose production was highly dependent on state-subsidized chemical fertilizers. Production of major crops such as rice and tea reduced drastically. This came on top of reduced demand due to the COVID-19 pandemic. In the COVID-19 recovery phase, agricultural exports were still low, but Sri Lanka’s own food security was hurt badly, as food prices spiked – forcing the country to import major food crops such as rice.
Infrastructure Rush and Environmental Fragility
Sri Lanka has defaulted on US$ 51 billion external debt. It has also borrowed from various multilateral organisations such as the World Bank, International Monetary Fund, and Asian Development Bank. However, the Chinese Belt and Road Initiative (BRI) in particular injected an impetus into large infrastructure projects, often called the “ white elephant projects ”, as they are offered at higher interest rates, provided low returns, in addition to not benefitting the local population. These large-scale infrastructure projects have also added to environmental disruptions and pressures.
For instance, The Hambantota project and the Colombo Port City project have been the focal points of several protests by the local communities against the government. The Chinese investments on the island, in particular, have led to diversion of agricultural lands, cutting of shrub jungle, and disruption of elephant corridors. The lack of transparency around these projects and their environmental and social impacts have raised serious concerns, especially since the Sri Lankan government went ahead with the project approvals without enough public consultations or risk due diligence. In the case of Hambantota project, apart from environmental risks such as landslides and coastal erosion, the lack of environmental impact assessments has already been blamed for flooding in the area in the past.
A Shipping Disaster
Sri Lanka’s geographical location – lying at the centre of the Indian Ocean shipping lanes – is yet another factor that adds to its vulnerability (as much as it could boost its economic prospects). In 2021, a container ship (‘MV X Press Pearl’) allegedly carrying “ 25 tonnes of nitric acid , 348 tonnes of oil,” and “up to 75 billion” of nurdles (plastic-particle water pollution), caught fire and sank off the coast of Colombo. The real impacts of this disaster are still unknown, as various national and international agencies have failed at measuring the cost and extent of environmental damage. In addition, the disaster response itself has been an expensive affair, as Sri Lanka’s clean-up mission on its shores and beaches are far from over.
This disaster may not be in the news now, but has long-term consequences for a country already grappling with current unrest. In a country, where close to 5 million people are dependent on coastal and marine fisheries for their livelihoods, the dangers posed by this disaster to marine biodiversity and future disaster risks, coupled with overfishing and illegal fishing , destruction of marine and coastal habitats, pollution, and climate change, are immense.
The fishing communities , already affected by the COVID-19-related lockdowns, were further struck by the ban on fishing in the aftermath of this disaster, leading to further economic and livelihood losses for these communities. This is yet another case of systemic and compound risks that have worsened the crisis in Sri Lanka further.
Russia-Ukraine Crisis
The Russia-Ukraine crisis has aggravated Sri Lanka’s environmental and socio-political fragilities. Like many developing countries, Sri Lanka is dependent on wheat, cooking oil, and fertilizer imports from Russia and Ukraine. In addition to the debt crisis, the war in Ukraine has led to hikes in food, energy, and consumer prices in the country, while creating severe shortage of several commodities, especially fuel. Amidst the brewing crisis, the country has also been struck by a heatwave . Finally, since the economic crisis worsened, many Sri Lankans have been trying to flee the country, legally or illegally, crossing dangerous oceanic waters.
According to a United Nations survey , “some 70 per cent of Sri Lankan households reported cutting back on food consumption, with food price inflation running at around 57 per cent.” With long queues at fuel stations and extended power cuts (coupled with the lack of assistance offered by the G-7 countries), Sri Lanka is looking to buy crude oil from Russia at a cheaper rate. With this crisis, Sri Lanka’s fossil fuel dependence is further entrenched, with a risk of also falling foul of secondary Western sanctions.
The Way Forward for Sri Lanka is Shaky
Any efforts to bring normalcy and ensure stability on the island nation should entail integrated risk planning, which clearly acknowledges and targets the structural risks mentioned above. While the immediate priority would be to alleviate the suffering of the Sri Lankan people by providing aid (food, fuel, etc.), the scale of the crisis is too big to be resolved within a short span of time.
International donors, including European ones, could consider assisting the country reduce dependence on fossil fuel imports and develop renewable energy infrastructure in a sustainable and participatory manner. One of the solutions could be to invest in decentralized renewable energy systems that ensure access to affordable energy to all. Considering that sectors such as tourism are already adversely affected by the COVID-19 pandemic and now the ongoing political crisis, it could be rejuvenated by investments in sustainable tourism , focussing on biodiversity protection, climate adaptation, and socio-economic well-being. Similarly, in the agricultural sector, lessons learnt from the organic farming debacle could be used to roll out measures to re-introduce organic and regenerative practices in a phased manner.
This article was originally published on planetarysecurityinitiative.org .
Related content
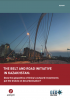
The Belt and Road Initiative - The case of Kazakhstan
The Belt and Road Initiative (BRI) is a prime example of how decarbonisation processes interact with geopolitics. The successful implementation of the Paris...
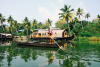
Can South Asian rivers be ‘regional public goods’?
A multi-sectoral and multilateral approach to South Asia's rivers could provide sustainable development, but it needs to include those already marginalised by a...
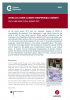
China as a new climate-responsible donor?
China is rapidly evolving into one of the world’s largest overseas investors and is now increasingly investing in the renewable energy sector. China has also...
- Climate Diplomacy
Asian Development Bank signals end to ‘dirty’ coal finance
As falling renewable energy costs and a shadow carbon price are making coal power investments unviable the Asian Development Bank (ADB) is making a decisive...
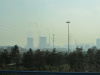
Polluting investments? Why we need a new era of development cooperation
As opposed to China’s Belt and Road Initiative, India could lead a campaign for climate-responsible international development cooperation, shifting from coal to...
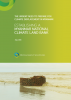
- Adaptation and Resilience
The urgent need to prepare for climate displacement in Myanmar: Establishing a Myanmar National Climate Land Bank
A new report released in May by Displacement Solutions and Yangon-based Ecodev urges the government of Myanmar to immediately establish a Myanmar National...
Regional initiatives can be a milestone for addressing planetary security
The destruction caused by Cyclone Ockhi in South Asia portends what a ‘climate-changed’ world has in store for humankind, especially taking into consideration...
Energy, Climate Change and Poverty: Insights from a Field Trip to Odisha, India
Vigya Sharma travelled to the state of Odisha, on India’s east, to get some insights on the linkages between energy access, rural poverty and climate change...
- Sub-Saharan Africa
- Conflict Mitigation and Peacebuilding
Ethnic diversity and climate change: lessons on the conditions that bring about conflict
A paper published last week in Proceedings of the National Academy of Sciences tests the hypothesis that climate related natural disasters may be part of the...
Environmental Pollution And Its Challenges In Sri Lanka
- DSpace Home
- Research Awards
- Faculty of Technology
- 2019 - Technology
Show simple item record
Files in this item
This item appears in the following collection(s).
- 2019 - Technology [29]
Search DSpace
All of dspace.
- Communities & Collections
- By Issue Date
This Collection
Thank you for visiting nature.com. You are using a browser version with limited support for CSS. To obtain the best experience, we recommend you use a more up to date browser (or turn off compatibility mode in Internet Explorer). In the meantime, to ensure continued support, we are displaying the site without styles and JavaScript.
- View all journals
- My Account Login
- Explore content
- About the journal
- Publish with us
- Sign up for alerts
- Open access
- Published: 20 October 2024
Estimating dynamics of dengue disease in Colombo district of Sri Lanka with environmental impact by quantifying the per-capita vector density
- Piyumi Chathurangika 1 ,
- S. S. N. Perera 1 &
- S. A. Kushani De Silva 1
Scientific Reports volume 14 , Article number: 24629 ( 2024 ) Cite this article
454 Accesses
6 Altmetric
Metrics details
- Applied mathematics
Dengue is a vector-borne disease transmitted to humans by vectors of genus Aedes causing a global threat to health, social, and economic sectors in many of the tropical countries including Sri Lanka. In Sri Lanka, the tropical climate, marked by seasonal weather primarily influenced by monsoons, fosters optimal conditions for the virus to spread efficiently. This heightened transmission results in increased per-capita vector density. In this work, we investigate the dynamic influence of environmental conditions on dengue emergence in Colombo district − the geographical region with the highest recorded dengue threat in Sri Lanka. An iterative approach is employed to dynamically estimate dengue cases leveraging the Markov chain Monte Carlo simulations, utilizing the dynamics of four seasons per year influenced by monsoon weather patterns governing in the region. The developed algorithm allows to estimate the risk of dengue outbreaks in 2017 and 2019 with high precision, facilitating accurate forecasts of upcoming disease emergence patterns for better preparedness. The uncertainty quantification not only validated the accuracy of outbreak estimates but also showcased the model’s capacity to capture extreme cases and revealed undisclosed external factors such as human mobility and environmental pollution that might affect dengue transmission in the Colombo district of Sri Lanka.
Similar content being viewed by others
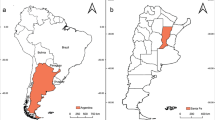
Dengue emergence in the temperate Argentinian province of Santa Fe, 2009–2020

Bayesian spatio-temporal analysis of dengue transmission in Lao PDR

Epidemiological characteristics and transmission dynamics of dengue fever in China
Introduction.
Dengue fever is an infectious disease caused by the vector-borne virus (serotypes DENV-1 to DENV-4) transmitted by the mosquitoes of the species Aedes 1 . It is a global health issue, with an estimated occurrence of 100 million cases annually across the globe. The dengue cases have increased in recent years, 30-fold over the past 50 years with a 50% increase in the past decade alone, with a growing number of countries reporting outbreaks 2 , 3 , 4 , 5 . In 2020 alone, over 2.5 million cases of dengue were reported worldwide with 1,018 (0.04%) deaths 6 . The World Health Organization (WHO) has declared dengue fever an endemic in over 100 countries, most of which are in tropical and subtropical regions of the world, including Southeast Asia, South America, and parts of Africa. The dengue threat is highest in Asia and the Americas, with Southeast Asia and the Western Pacific region accounting for over \(70\%\) of the global dengue statistics. In Sri Lanka, dengue threat is the highest in the Western province reporting frequent outbreaks 7 . The most recent outbreaks were reported in 2017 and 2019. There were over 51,000 cases, with over 90 deaths (0.17%) reported during the 2017 outbreak and 186,101 cases with over 440 (0.23%) deaths were reported during 2019 outbreak according to the Epidemiology unit, Ministry of Health, Sri Lanka. In both of these instances, the Sri Lankan health system collapsed due to unpreparedness and lack of optimum resource allocations. The Sri Lankan government has implemented various health and social measures to prevent and control the spread of the disease by conducting public awareness campaigns, clean-up programs to remove mosquito breeding sites, insecticide fumigation and increasing the use of mosquito repellents 7 , 8 . However, similar to other tropical countries, Sri Lanka too has failed to completely wipe-out or successfully control the disease emergence. Given the heightened risk of infectious diseases in the future as a consequence of climate change, it’s essential to anticipate forthcoming dengue threats. This enables adequate time for bolstering preparedness and strengthening the healthcare system.
The infected human and vector population densities are intricately linked to the intensity of virus transmission 9 . The Aedes vectors thrive in warm and humid environments commonly found in tropical and subtropical regions. In particular, Aedes mosquitoes have multiple stages in their life cycle - egg, larva, pupa, and adult. Mosquitoes lay their eggs in stagnant clean water sources such as uncovered containers, discarded tires, and water-filled areas. With an abundant supply of breeding sites and favorable climatic conditions, the vector population can grow rapidly. Consequently, this high vector population density can amplify the likelihood of dengue transmission for several reasons such as (1) a larger vector population means a greater chance of female vectors being infected with the dengue virus, (2) more mosquitoes increase the probability of contact between infected vectors and susceptible individuals enabling the virus to be transmitted more rapidly 10 . Therefore, the vector population significantly impact the dengue transmission within human populations. Environmental conditions directly impact the mosquito population density and therefore can create high sensitivity in dengue transmission 7 , 11 , 12 , 13 , 14 , 15 , 19 . The warmer and humid environments, typically between 26−30 °C (77−86 °CF) of temperatures and above 60% of humidity levels provide a highly favorable environment for the Aedes mosquitoes causing rapid transmission 16 , 17 , 18 . Sri Lanka experiences an average temperature between 26−30 °C, with coastal regions being slightly warmer than inland areas. The humidity level remains consistently above 70% throughout the year reaching nearly 90% during the monsoon season in June. Monsoon rainfalls in Sri Lanka directly impact the dengue emergence patterns, as these rains create ideal breeding conditions for Aedes mosquitoes 19 given the favorability of the other environmental conditions 20 , 21 , 22 . Specifically, Sri Lanka falls within the highest-risk category for epidemic potential according to the projected climate change 11 . Apart from favorable and consistent temperature and humidity conditions throughout the year, rainfall from the two monsoons experienced by Sri Lanka adds an additional level of favorability, generating high risk of dengue 23 , 24 , 25 . In addition to the influence of rainfall, the increased density of hosts and vectors, along with their mobility, contributes to the heightened transmission rate.
Given the severity of the disease, timely forecasting its future risk is critically important to alleviate the stress on the Sri Lankan health system. Moreover, the control measures such as early detection of rapid development, diagnostics and surveillance measures are vital for better handling the dengue threat 26 . Due to the influence of external factors on vector populations and disease transmission, disease control strategies may not be universally applicable. Tailoring approaches based on specific geographic locations and timeframes will be crucial for achieving effective and efficient outcomes 19 . Numerous studies have delved into modeling and validating intricate relationships between weather patterns and the emergence of dengue, employing various methodologies. Particularly models developed connecting information of weather and dengue in Sri Lanka are concentrated to establishing the correlation between these variables followed by regression or time-series models 27 , 28 , 29 , 30 , 31 , 32 . Additionally, the intricate dynamics of disease transmission between vectors and humans are extensively explored through compartmental models. These models depict the vector-host relationship by ordinary differential equations to characterize the population dynamics of both entities 33 , 34 , 35 , 36 , 37 . Typically, the human population is compartmentalized into three categories: susceptible ( \(S_h\) ), infected ( \(I_h\) ), and recovered ( \(R_h\) ), while the vector population is often divided into susceptible ( \(S_v\) ) and infected ( \(I_v\) ). Of these models, disparity in population dynamics between vectors and humans as time progresses was notable. While human populations may exhibit relatively gradual changes, vector populations can undergo drastic fluctuations within short time intervals due to environmental conditions when combined with their short life span. However, these models assume the infection dynamics progress more rapidly for humans than for the vector, achieved by mathematically approximating the system of equations at its quasi-equilibrium of vectors (i.e., \(\dot{I_v}=0\) ). While this simplification results in a more straightforward model, it overlooks the potential impact, if any, of changes in the vector population on the infection dynamics of humans 38 , 39 . Consequently, vector population variations in response to external factors, particularly weather data, are often overlooked. This oversight of the models can lead to significant limitations in the estimations and simulation processes, as they fail to capture the comprehensive picture of the epidemic. The neglected variations in vector populations with respect to weather data results in a gap in understanding the full impact of environmental factors on disease transmission dynamics. As a result, the insights gained from these models may not accurately reflect the complexities inherent in the epidemic. To address the existing gap in estimating dengue risk based on fluctuating vector populations in the Colombo district, our study aims to establish a quantitative relationship between vector density and a key environmental factor specific to the study region. Specifically, we do not assume a constant vector population in the ordinary differential equation system. Instead, we introduce an indirect optimization approach to estimate vector density in the Colombo district. This method provides a more targeted and accurate estimation of vector populations, leading to improved predictive capabilities.
While several experimental studies have investigated vector populations across different regions of Sri Lanka, the findings from these studies are not universally applicable due to the varying environmental conditions, socio-economic factors, and unique characteristics of each region. As a result, these experimental data, although valuable, are highly case-sensitive and cannot be generalized across the country. Moreover, conducting such experimental studies is often costly and time-consuming, limiting their feasibility on a broader scale. However, our study seeks to develop a method which will indirectly estimate vector densities by correlating to rainfall, offering a more efficient and indirect approach. Accordingly, our study aims to close these gaps related to vector population by establishing a measurable link between weather patterns and the dynamics of vector populations, thereby improving our comprehension of the factors influencing the emergence of dengue. As outlined in literature models for Sri Lanka, rainfall significantly influences the occurrence of dengue cases. When examining the impacts of minimum temperature, maximum temperature, humidity, and rainfall on dengue, principal component regression analysis has demonstrated rainfall accounts for over 95% of the influence on dengue cases in Colombo district. In existing literature, environmental variables are typically used directly to estimate vector populations through regression-type functions. However, we introduce a novel approach that utilizes optimization via Markov chain Monte Carlo (MCMC) to infer vector populations by optimizing the impact of rainfall, leveraging existing data on human infection incidences. In particular, we developed a model based on a probabilistic and dynamical approach connecting rainfall to estimating the per-capita vector density in a SIR-SI (Susceptible, Infected, Recovered for human - Susceptible, Infected for vector) model of differential equations. Moreover, given the compelling evidence of rainfall’s impact on dengue, estimations are performed within identified seasons to align with the monsoon-induced rainfall patterns. Therefore, the estimation is demonstrated in an iterative process for each season in a feedback loop. This estimation algorithm based on Bayesian methods enabled uncertainty quantification using credible intervals for each season. Conducted in the hotspot of dengue emergence, which reports the highest number of dengue cases in Sri Lanka, this study provides critical insights for health authorities to enhance prevention and mitigation strategies.
Method and analysis
The highly densed population in the Colombo district continuously accounts for the highest number of reported dengue cases in the country. Within this district, Colombo Municipal Council (CMC) area stands out for its highest population density, with 24,857 residents per \(km^2\) , making it the most densed region in the Colombo district 40 , 41 . Monsoons, southwest monsoon from May to September with average total rainfall 2500-4000 mm and the northeast monsoon from December to February with average total rainfall 1000−2000 mm, are one of the major origins of the rainfall patterns in Sri Lanka 20 , 42 . The Colombo district, situated in an agricultural wet zone, experiences greater benefits from the southwest monsoon compared to the northeast, primarily because of its geographical positioning (see Fig. 1 (b)). Dengue incidences in Sri Lanka is found to be governed by the seasonal rainfall variations experienced throughout the year (see Fig. 1 ). Based on these rainfall variations, dengue emergence seasons can also be identified by calculating the periodicities of the reported dengue incidences. These periodicities of dengue and rainfall are quantified with Fast Fourier Transform (FFT). Fig. 2 depicting FFT amplitudes indicates both rainfall and dengue exhibit their peak levels at 6-month cycles (see Supplementary Material for numerical results). With these results of 6-month periodicities, a year is divided into 4 segments, which are referred to as seasons throughout the paper. The time span of a season was identified based on the increasing and decreasing trends in the reported rainfall fluctuations and a total of \(s=54\) rainfall seasons were identified during the period of 2009 January and 2022 December. Consequently, the number of weeks comprising each season may differ resulting in distinct durations. Moreover, the impact of rainfall on dengue emergence is studied through wavelet analysis 25 , 46 , 47 . In particular, a \(10 -\) week time lag has been identified between rainfall and dengue emergence in the CMC area.
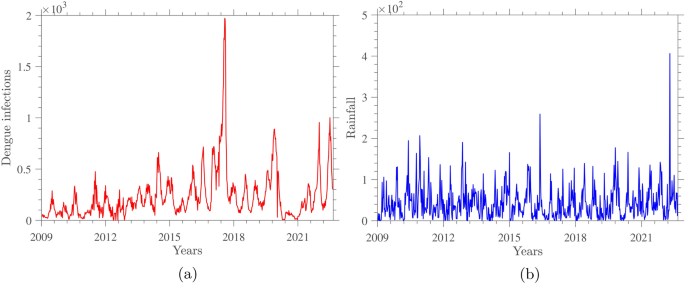
Variations of dengue infections and rainfall. Variation of weekly ( a ) dengue infected human population (confirmed dengue infected patient count obtained from the National Dengue Control Unit (NDCU), Ministry of Health, Sri Lanka) and ( b ) rainfall in the CMC area from 2009−2022 (data obtained from the NASA power data access viewer 48 ).
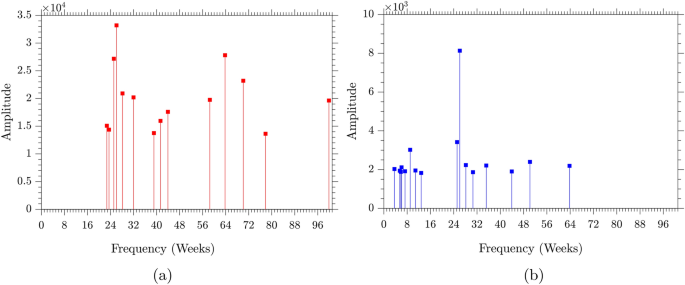
Periodicity of dengue and rainfall variations. Amplitudes are highest for 26 weeks in both ( a ) dengue (amplitude is 8133.34) and ( b ) rainfall (amplitude is 33192.65).
This study uses a SIR- SI compartmental model where human population has three epidemiological states - susceptible ( \(S_h\) ), infected ( \(I_h\) ), recovered ( \(R_h\) ), and vector population has two such states - susceptible ( \(S_v\) ) and infected ( \(I_v\) ) (see Fig. 3 ). Vectors have no recovery state since they do not survive long enough to undergo recovery from the disease. It is assumed the compartments respectively belonging to human and vector preserve a closed system, i.e. the populations are constant ( \(N_h={S}_h+{I}_h+{R}_h\) and \(N_v={S}_v+{I}_v\) ). One of the limitations of this study is the lack of data availability on distributions of Aedes species and their serotypes. As a result, to setup our model we assumed a homogeneous distribution for both serotype and species. The ordinary differential equations (ODE) system of five state variables is given in Supplementary Material. This five state variable model is reduced to three state variables \({I}_h, {R}_h, \text { and }{I}_v\) ( \(3-D\) ) using the assumption of closed populations (see Appendix 1 in Supplementary Material). Further, this \(3-D\) population model is converted to \(3-D\) density model by normalizing the state variables, i.e. \({I}={{I}_h}/{N_h}\) , \({R}={{R}_h}/N_h\) , \({V}={{{I}_v}}/{N_v}\) . The reduced ODE system of I , R , and V is given in model ( 1 ). The definitions of model ( 1 ) parameters are given in Table 1 .
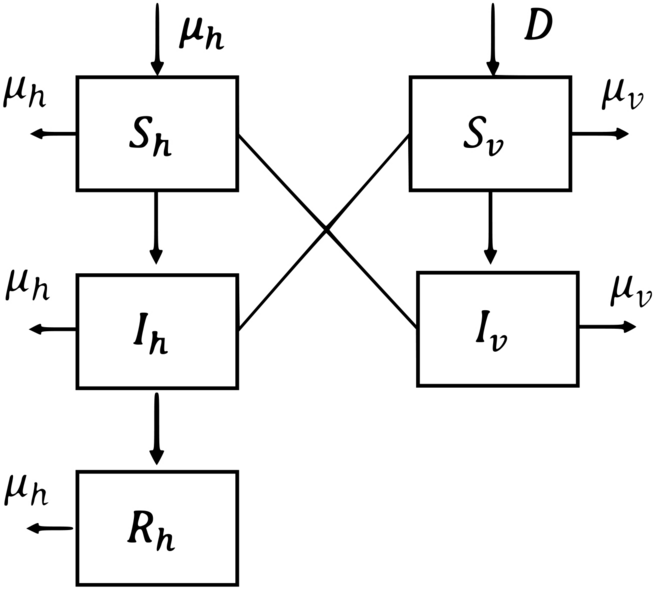
Schematic diagram of transmission of the dengue virus between human (host) and mosquito (vector) populations. \(S_h\) , \(I_h\) , and \(R_h\) are the susceptible, infected, and recovered host populations respectively. \(S_v\) and \(I_v\) are the susceptible and infected vector populations respectively.
Of the parameters in model ( 1 ), the ratio \({N_v}/{N_h}\) is defined as the per-capita vector density ( z ), which denotes the total number of vectores available per human. z is highly dependent on abundant vector breeding sites after rainfall 21 , 22 , 25 . Hence, we assume the vector population is directly proportional to the rainfall patterns (see Eq.( 2 )), since other environmental variables such as temperature and humidity are favorable to vector breeding throughout the year 19 , 21 , 22 . In this context, we presume a linear relationship due to the absence of evidence warranting the introduction of complexities inherent in a nonlinear model. Given the rainfall seasonality originated from monsoons we establish the relation in Eq.( 2 ) seasonally. From this point onward, the bold symbols such as \(\textbf{E}\) represent vectors and non-bold symbols represent scalars. These vectors may vary in length for each season (see Supplementary Material).
where, for the \(i^{th}\) season, \(\textbf{E}_i\) is the reported weekly total rainfall, \(a_i\) is the rainfall coefficient, and \(\textbf{z}_i\) is the per-capita vector density. The simulation of model ( 1 ) for a season requires estimating \(\textbf{z}\) using rainfall, which in turn demands estimating a . We infer this parameter a for each season in an iterative process. The estimation procedure in Bayesian framework yields,
where \(\theta\) and x respectively denote the parameters and data. Here K is the normalization constant of the posterior probability distribution. In this problem, parameters (unknowns to be estimated) and data (observed information) are identified in Table 2 . In parameter estimation, the actual dengue density data ( \(\mathbf {I_{obs}}\) ) are compared against the solutions of the ODE system ( \(\hat{\textbf{I}}\) ) in Eq.( 1 ). Weekly reported dengue cases in the CMC area were obtained from the National Dengue Control Unit (NDCU) of Sri Lanka. We acknowledge that reported dengue incidences may not present the full spectrum of total infections. However, the consistency and rigor with which these reports are compiled by the NDCU provide a strong foundation for our analysis. The rainfall data required for this study were extracted from 48 . The remaining parameters in the model ( \(\textbf{P}\) ) were borrowed from the literature and assumed constant for every season 49 , 50 (see Table 2 ). The borrowed parameter values for the simulation are provided in the Supplementary Material.We borrowed values from the literature due to the absence of experimental data specific to the Colombo district. Furthermore, we have assumed there is no significant change in these values during the study period, allowing us to utilize fixed values throughout the analysis.
Next we demonstrate the parameter estimation setup for a season and for brevity we eliminated the subscript i , which denotes seasons. The parameters and data applied to the model in ( 3 ) yields,
where \(\mathscr {I}\) denotes any background information according to the standard Bayesian setup. The error variance \(\sigma\) is sampled, independently from the Bayesian setup, using inverse gamma distribution and it is explained in the next section. There is no existing knowledge for the rainfall coefficient or the rainfall and thus we assume uniform priors for \(p\left( a|\textbf{E}, \mathscr {I}\right)\) and \(P(\textbf{E}|\sigma ,\mathscr {I})\) . The log likelihood function, assuming errors in observed data follows Gaussian distribution yields,
where \(||.||^2\) denotes the L 2 norm of the errors and \(\hat{\textbf{I}}=\displaystyle \int \dfrac{d\textbf{I}}{dt}dt\) . Finally the log posterior distribution yields,
We then encapsulated this Bayesian posterior simulation process into the Alg. 1 showcasing the iterative procedure of parameter estimation for s number of seasons. The optimization problem is a \(\text {Min}_{a\in \mathbb {R}} \log p\left( a | \mathbf {I_{obs}},\textbf{E}, \textbf{P},\mathbf {Y_0},\sigma , \mathscr {I}\right)\) .
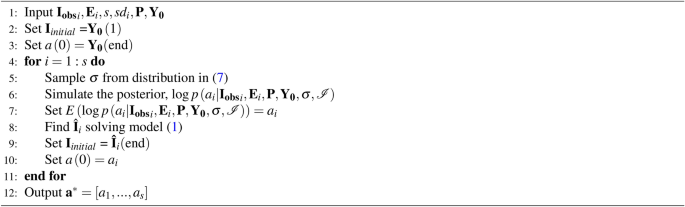
Estimating rainfall coefficients, \(a_i,\quad i=1,\cdots ,s.\)
The marginal posterior distributions obtained for each seasonal rainfall coefficient parameter enable us to calculate the 95% credible intervals. These intervals provide a statistical range for each parameter, reflecting the uncertainty in our dengue incidence estimates. The sensitivity of rainfall coefficient estimates to the dengue incidence estimates are given by the credible intervals calculated from the uncertainties of rainfall coefficients and estimated dengue densities from the ODE model ( 1 ). Sensitivity of the parameters allow us to better understand the variability and reliability of our seasonal estimations and predictions. The model validation can be captured via estimates of dengue incidences during specific seasons, for example outbreak periods or festive seasons.
Results and discussion
The posterior distribution in Eq. ( 6 ) was simulated using MCMC toolbox of Delayed Rejection Adaptive Metropolis (DRAM) in MATLAB 2024a 51 , 52 . The MCMC algorithm systematically explores sample values for the rainfall coefficient \(a_i\) , from a chosen probability distribution, which resulted in a series of random samples. For each of these sampled values of \(a_i\) , the algorithm computes the corresponding output for \(\mathbf {\hat{I}}\) and compares it to the observed dengue incidences ( \(\mathbf {I_{obs}}\) ). We then choose the optimum parameter value from the sampled \(a_i\) values resulting the least error between \(\mathbf {\hat{I}}\) and \(\mathbf {I_{obs}}\) out of the sampled \(a_i\) values. This optimum \(a_i\) is then used to obtain the simulated \(\mathbf {\hat{I}}\) . In the simulations of model ( 1 ), the initial values of \(\mathbf {Y_0}\) were chosen respectively to be [ \(I_0,0,V_0, 10\) ]. Here \(V_0\) is calculated assuming the vector population is at its quasi-equilibrium. \(I_0\) is the initial value in reported dengue infected human population density. The extracted values for \(\textbf{P}\) were \(\beta _h= 0.75, \mu _h= \frac{1}{75\hspace{0.1cm} \text {years}}, \beta _v=0.375, \gamma _h = \frac{1}{2\hspace{0.1cm} \text {weeks}}\) and \(\mu _v = \frac{1}{6 \hspace{0.1cm}\text {weeks}}\) 49 , 50 . The MCMC toolbox, DRAM, samples error variance values \(\sigma\) using an inverse gamma distribution ( r , q ) for each season (via update sigma \(=1\) 51 ),
where \(r=(1+N)/2, q=2/(sd+SSE)\) with N , SSE respectively represent the sample size and the \(\Vert {\mathbf {I_{obs}} - \mathbf {\hat{I}}}\Vert ^2\) . Starting from the second season, the initial values, \(I_0,R_0,V_0\) , were extracted from the estimated solutions of the preceding season. Thus, the iterative estimations for all 54 seasons were performed solving the optimization problem in Alg. 1 using the MCMC simulations. For each season \(N_d=5\times 10^4\) simulations were sufficient to observe the convergence of the samples. With these obtained convergent samples \(30\%\) of initial samples were discarded for accuracy purposes and the marginal probability distributions were obtained for each seasonal parameter. Marginal distributions obtained for seasons 32, 50, 22, and 27 are depicted in Fig. 4 . The best point estimate of \(a_i\) is taken as the mean of the marginal distribution,
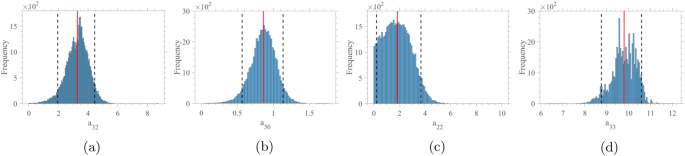
Marginal distribution simulations of rainfall coefficients. Histograms of the marginal distribution obtained for 4 seasons(a) 2017 January-April (b) 2021 April-July, (c) 2009 July-October, (d) 2017 April-July are given. The red vertical lines indicate the means of marginal distributions. The black dashed lines indicate the 95% credible intervals of each marginal distribution.

Dengue infection density estimates of first two seasons in (a) \(s_1\) and (b) \(s_2\) . The black circles indicate the reported dengue infected human population density and the red line indicates the estimated line. The fitted curve in (a) is obtained by taking the actual initial values at the beginning of the time period. The parameter value, \(a_1\) obtained for season 1 is 10.79. The fitted curve in (b) is obtained by taking the final values of the fitted curve in (a) as the initial values for season in (b). The parameter value, \(a_2\) obtained for season 2 is 2.13.
Figure 5 shows the solution curves of \(\mathbf {\hat{I}}\) against observed data ( \(\mathbf {I_{obs}}\) ) for the first two seasons. The uncertainties of these estimates were obtained by calculating the credible intervals at 95% level from the simulated marginal distributions of rainfall coefficients ( \(a_i\) ) (see Fig. 4 ). The estimates of rainfall coefficient ( \(a_i\) ) for all seasons are provided in Supplementary Material. The uncertainties of the rainfall coefficient allowed to obtain the uncertainties of the dengue densities, acknowledging the potential impact of undisclosed external variables. Figure 6 depicts the inferred infected population density ( \(\mathbf {\hat{I}}\) ) (red line) with their uncertainties (gray shades) for the whole study period. The results accurately captured the dengue outbreaks took place during 2017 (seasons \(32-35\) ) and 2019 (seasons \(40-43\) ) (see Fig. 6 ). In outbreak years the uncertainties are minimal, underscoring the precision of the estimates and the model’s ability to capture extreme cases when supported by evidence. These relatively narrow uncertainties in outbreak seasons compared to other seasons suggest rainfall is likely one of the primary factors contributing to these outbreaks. Our results effectively capture both the trends and seasonality in dengue incidences, revealing a strong correlation between dengue incidence patterns, fluctuations in vector density, and rainfall patterns. This highlights the significant impact of monsoon rainfall and its seasonality on the spread of dengue in the Colombo district. Consequently, seasonal variations in monsoon rainfall are likely to forecast corresponding seasonal variations in dengue incidences.
However, the estimated results show large uncertainties in specific seasons requiring further investigation. The highest uncertainties (width of the credible interval \(>10\) ) are observed in season 21 (month April) and season 31 (month December). The marginal distributions of these seasons show the possible existence of mix Gaussian distribution suggesting the impact of some external factors possibly overpowering the impact of rainfall (Fig. 4 (c)). Especially during the festive seasons in April and December, disease transmission elevates due to the increasing human mobility and population gatherings. The moderately high uncertainties are observed in seasons \(7,8,11,12,14-16,22,24,28,32,36,38,42,45,47,48\) , and 53 (width of the credible interval between 3 and 6). In addition, unexpected above-average rainfall incidences also may have an impact in the differences shown in the marginal distributions. Utilizing initial values, per-capita vector density, and rainfall data specific to a particular season, the algorithm consistently forecasted dengue cases.
By utilizing these parameters, one can forecast upcoming dengue emergence using the rainfall data. Thus in this study, an out-of-sample forecast was employed where dengue emergence is forecasted for a period of 10 upcoming weeks using rainfall data. The uncertainty of forecast is inferred in two ways (Fig. 6 green box): the initial values \(\mathbf {Y_0}\) for the algorithm are chosen (a) as the point estimates from the last season (the inner shaded area in Fig. 6 ) and (b) as the credible interval (lower and upper bound values) of the last season (the outer shaded area in Fig. 6 ).

Estimated dengue infected human population density in the CMC area. The red line shows the estimated results ( \(\mathbf {\hat{I}}\) ) (see Supplementary Material). The gray circles show the reported infected human population density values calculated from the reported patient count data obtained from the NDCU ( \(\mathbf {I_{obs}}\) ). Uncertainties of results at 95% level are shown by the shaded gray color bands. Forecast of dengue emergence for one upcoming season is given in the green box. The forecast was obtained in two different ways. The inner band (solid line) in the forecast shows the uncertainty of the forecast based on the end point of the estimated dengue density in the previous season. The outer band (dash line) shows the uncertainty of the forecast based on the end points of the uncertainties (lower and upper) of the dengue estimates in the previous season.
Given the ongoing challenges in dengue eradication, particularly due to the absence of a cure or widely available vaccine, understanding the emergence patterns and timing of dengue outbreaks becomes a cornerstone of effective control and prevention strategies. The insights gained from this study in understanding the dengue emergence patterns are indispensable for enabling public health authorities to implement timely interventions, such as fogging with insecticides or the release of Wolbachia -infected mosquitoes, both of which play crucial roles in reducing vector populations and interrupting the transmission cycle of the virus. Additionally, understanding the timing and intensity of rainfall is pivotal in issuing early warnings to the public, empowering households to take necessary actions to eliminate potential vector breeding sites. This is especially critical in areas like the CMC, where high human mobility and dense populations create a fertile environment for rapid dengue transmission. In such settings, the interplay between rainfall conditions and vector activity becomes a vital factor in forecasting dengue outbreaks, thus enabling timely decision-making and the deployment of targeted control measures. The implications of these findings underscore the need for a nuanced, context-specific approach to dengue control, one that integrates environmental monitoring with public health strategies to mitigate the impact of this persistent threat.
The findings of this study provide a foundation for developing a comprehensive early warning system for dengue emergence in the hotspots of Sri Lanka. By leveraging the observed correlations between rainfall patterns and vector population dynamics, an automated system could be designed to predict periods of increased vector activity and, consequently, heightened disease risk. This system would analyze rainfall data in real-time, using predictive models to forecast vector emergence and potential dengue outbreaks. Such a proactive approach would allow public health authorities to issue timely warnings and implement preventive measures well in advance, significantly mitigating the threat of dengue. For instance, our study’s findings could inform the development of an efficient vector control system by determining the optimal timing for cleaning and insecticide application in relation to rainfall patterns. By anticipating risk and acting before the disease spreads, this system could play a crucial role in reducing the incidence of dengue and protecting vulnerable communities across the country.
This study operates under several key assumptions regarding the emergence of dengue fever within the CMC area. Firstly, this study and its findings are specific to the Colombo district in Sri Lanka, and therefore have limitations when generalizing to other regions globally. However, this study presents a methodology that can be adapted to areas with unique weather patterns. Considering the region-specific findings, we outline the inherent limitations of this study, which can be refined in future research. It is assumed the primary vectors responsible for dengue transmission, Aedes aegypti and Aedes albopictus , and their serotype distribution, are homogeneously distributed throughout the region. Due to the lack of these data in Colombo district, our estimates may not be accurate and possibly represent in the estimated uncertainties. Further our estimates do not represent the intensities of cases due to species type or the virus serotype. The emergence of dengue in this area is hypothesized to be predominantly driven by rainfall, given that other environmental conditions, such as temperature and humidity, are consistently favorable for the breeding and survival of these vector species. Thus, any dengue incidences caused by unexpected fluctuations in environmental conditions other than rainfall are not reflected in our results. In the ODE system, both human and vector populations are assumed to be within a closed system, where the total number of humans and vectors remains constant throughout the study, with no significant external migration or population shifts. This assumption could influence our results, especially given Colombo’s status as a commercial hub with high human mobility. However, we were able to account for this during the Christmas/New Year period through the uncertainties. Additionally, the study assumes that parameters like transmission rates, drawn from existing literature, remain constant over time, without considering potential seasonal variations, which could influence our estimates. Another limitation of our study is the lack of experimental data for the model parameters. Using actual values reflecting dengue transmission in the Colombo district could improve the accuracy of our results. However, these type of errors are usually captured by the uncertainty of results and therefore our estimates account for the possible errors in the chosen parameter values. Additionally, while we have configured our model using fixed parameter values, it would be advantageous to employ dynamic parameters for factors such as transmission rates, though this would add complexity.

Conclusions
In conclusion, the findings of this study underscore the significant impact of weather variability, particularly monsoon rainfall patterns, on the ecology of vectors and the transmission dynamics of dengue in Sri Lanka. By integrating real-time rainfall data into disease mathematical model, our study introduced an algorithm to forecasting dengue cases taking into account the changes in vector populations. In that approach, we were able to estimate dynamic surge in vector abundance and consequently the infected human dynamics. Through this study, we successfully established a robust relationship between vector population dynamics and rainfall patterns. By elucidating how fluctuations in rainfall influence the breeding habitats of disease vectors, we gained valuable insights into the mechanisms driving dengue transmission. Leveraging this understanding, we developed a predictive model that not only quantified changes in vector populations but also enabled us to forecast dengue occurrences. Our study focuses on the Colombo Municipal Council (CMC) area, known for its high population density and significant dengue burden compared to other regions in the country. Our algorithm closely mirrored recorded dengue cases, accurately capturing outbreaks, particularly those in 2017 and 2019. Leveraging Bayesian methods, we quantified the uncertainty of our estimates (Fig. 6 ). While outbreaks exhibited low uncertainty indicating accurate predictions and the model’s capacity to capture extreme cases when supported by evidence, some seasons displayed large uncertainties suggesting the influence of undisclosed external factors apart from rainfall.
Upon careful observation the inferred high uncertainties are recorded in many of the seasons following Christmas/New Year times. Colombo Municipal Council (CMC) area being the commercial city of the country, Christmas and New year makes it the busiest and the most crowded city during these festive times. Due to the high population density with their frequent inbound and outbound mobility during the festivities, the city’s environmental pollution expected to elevate significantly. Thus during these seasons, the impact of rainfall is overpowered verifying the marginal distribution with high uncertainty. Other seasons with large uncertainties are reported following the seasons having above-average rainfall. Hence, it can be concluded, the external factors may dominate the impact of rainfall and may produce uncertainties in the forecast.
In summary, the iterative algorithm introduced in this study aims to estimate seasonal per-capita vector density and dengue cases using a probabilistic and dynamic modeling approach. By validating against real data and quantifying uncertainty, our approach demonstrates its effectiveness in precisely identifying outbreak years. Moreover, the uncertainty quantification reveals further insights into the elevated risk of dengue, emphasizing the necessity for continued exploration in future studies.
Data availability/Availability of data and materials
The data that support the findings of this study are available from the National Dengue Control Unit (NDCU), Ministry of Health, Sri Lanka, but restrictions apply to the availability of these data, which were used under license for the current study, and so are not publicly available. Data are however available from the author, Piyumi Chathurangika upon reasonable request and with permission of the National Dengue Control Unit (NDCU), Ministry of Health, Sri Lanka.
Halstead, S. B. Pathogenesis of dengue: challenges to molecular biology. Science 239 (4839), 476–481. https://doi.org/10.1126/science.3277268 (1988).
Article ADS PubMed Google Scholar
Bhatt, S. et al. The global distribution and burden of dengue. Nature 8 , 504–507. https://doi.org/10.1038/nature12060 (2013).
Article ADS Google Scholar
Guzman M., Halstead S., Artsob H.,Buchy P., Farrar J., Gubler D., Hunsperger E., Kroeger A., Margolis H., Martínez E., et al., Dengue: a continuing global threat. Nature reviews microbiology [SPACE] https://doi.org/10.1038/nrmicro2460 .
World Health Organization. Communicable disease threats report: Week 11, 12–18 March 2023 (World Health Organization, Weekly bulletin, 2023).
Siddique A. B. , Omi N. T., Rasel S. M.,Hoque S. S. B. ,Rahman N.,Sarker S., Ghosh A.,Ahmed I.,Akash Y., Ahmed A., et al., Assessment of perceived dengue risk and prevention practices among youth in Bangladesh. Scientific Reports 14 (1) (2024).
World Health Organization (WHO), Dengue and severe dengue, accessed: April 14, 2023 https://www.who.int/news-room/fact-sheets/detail/dengue-and-severe-dengue (2021).
Ministry of Health. Sri Lanka (National Dengue Control Programme, Ministry of Health, Sri Lanka, 2019).
National Dengue Control Unit, Ministry of Health, Nutrition and Indigenous Medicine, Sri Lanka, National action plan on prevention and control of dengue 2019-2023 (2019).
Padmanabha H., Durham D., Correa F., Diuk-Wasser M.,Galvani A., The interactive roles of Aedes aegypti super-production and human density in dengue transmission. PLoS Neglected Tropical Diseases 6 [SPACE] https://doi.org/10.1371/journal.pntd.0001799 (2012).
Focks D., A review of entomological sampling methods and indicators for dengue vectors. World Health Organization (2004).
Liu-Helmersson J., Stenlund H.,Wilder-Smith A. et al., Vectorial capacity of Aedes Aegypti: Effects of temperature and implications for global dengue epidemic potential. PLoS ONE 9 (3) [SPACE] https://doi.org/10.1371/journal.pone.0089783 (2014).
World Mosquito Program, How climate change is amplifying mosquito borne diseases (2019).
Naish, S. et al. Climate change and dengue: a critical and systematic review of quantitative modelling approaches. BMC infectious diseases 14 (1), 1–14. https://doi.org/10.1186/1471-2334-14-167 (2014).
Article Google Scholar
Leung, X. Y. et al. A systematic review of dengue outbreak prediction models: Current scenario and future directions. PLOS Neglected Tropical Diseases 17 (2), e0010631 (2023).
Article PubMed PubMed Central Google Scholar
Ong S. Q., Isawasan P., Ngesom A. M. M., Shahar H., Lasim A. m. M., Nair G., Predicting dengue transmission rates by comparing different machine learning models with vector indices and meteorological data. Scientific reports 13 (1) 19129 (2023).
Tjaden N. B. , Thomas S. M., Fischer D., Beierkuhnlein C., Extrinsic incubation period of dengue: knowledge, backlog, and applications of temperature dependence PLoS Neglected Tropical Diseases 7 (6) e2207. https://doi.org/10.1371/journal.pntd.0002207 (2013).
Wang, X., Tang, S., Wu, J., Xiao, Y. & Cheke, R. A. A combination of climatic conditions determines major within-season dengue outbreaks in Guangdong Province. China Parasites & vectors 12 (1), 1–10. https://doi.org/10.1186/s13071-019-3295-0 (2019).
Robert M. A., Christofferson R. C., Weber P. D., Wearing H. J., Temperature impacts on dengue emergence in the United States: Investigating the role of seasonality and climate change Epidemics 28 (9) [SPACE] https://doi.org/10.1016/j.epidem.2019.05.003 (2019).
Liyanage. P., The Influence of Climate and Public Health Interventions on Aedes Vectors and Dengue in Sri Lanka, Umea universitet (2022).
World Bank Group, Sri Lanka Climate Change Knowledge Portal Profile. Climate Change Knowledge Portal. (2020).
Sirisena, P., Noordeen, F., Kurukulasuriya, H., Romesh, T. A. & Fernando, L. Effect of climatic factors and population density on the distribution of dengue in Sri Lanka: A GIS based evaluation for prediction of outbreaks. PLoS ONE 12 (1). https://doi.org/10.1371/journal.pone.0166806 (2017).
Pratchaya, Chanprasopchai I. & M. T. Puntani Pongsumpun, Effect of rainfall for the dynamical transmission model of the dengue disease in Thailand. Computational and Mathematical Methods in Medicine [SPACE] https://doi.org/10.1155/2017/2541862 (2017).
Li, C., Lim, T., Han, L. & Fang, R. Rainfall, abundance of Aedes Aegypti and dengue infection in selangor, malaysia. The Southeast Asian journal of tropical medicine and public health 16 (4), 560–568 (1985).
PubMed Google Scholar
Naqvi S. A. A., Jan B., haikh S., Kazmi S. J. H., Waseem L. A., Nasar-u minAllah M., Abbas N., Changing climatic factors favor dengue transmission in lahore, pakistan. Environments 6 (6) (2019).
Chathurangika P., De. Silva K., and Perera S. S. N.. Dengue disease prediction with seasonality of environmental factors. , Communications in Combinatorics, Cryptography & Computer Science , 1 29–38, (2023).
Tambo, E., Chen, J. H., Zhou, X. N. & Khater, E. I. Outwitting dengue threat and epidemics resurgence in asia-pacific countries: strengthening integrated dengue surveillance, monitoring and response systems. Infectious diseases of poverty 5 (1), 1–5. https://doi.org/10.1186/s40249-016-0148-3 (2016).
Faruk, M., Jannat, S. & Rahman, M. S. Impact of environmental factors on the spread of dengue fever in Sri Lanka. International Journal of Environmental Science and Technology 19 , 10637–10648. https://doi.org/10.1007/s13762-021-03905-y (2022).
Edirisinghe. G, Contribution of rainfall patterns for increased dengue epidemic in Sri Lanka. American Scientific Research Journal for Engineering, Technology, and Sciences (ASRJETS) 35 (1) 284–294 (2017).
Sun, W., Xue, L., & Xie, X. Spatial-temporal distribution of dengue and climate characteristics for two clusters in Sri Lanka from 2012 to 2016. Scientific Reports 7 , (2017). https://doi.org/10.1038/s41598-017-13163-z .
Ehelepola, N., Ariyaratne, K., Buddhadasa, W., Ratnayake, S. & Wickramasinghe, M. A study of the correlation between dengue and weather in kandy city, Sri Lanka (2003–2012) and lessons learned. Infectious Diseases of Poverty 4 , 1–15. https://doi.org/10.1186/s40249-015-0075-8 (2015).
Withanage, G. P., Viswakula, S. D., Gunawardena, Y. I. N. S. & Hapugoda, M. D. A forecasting model for dengue incidence in the District of Gampaha. Sri Lanka. Parasites & vectors 11 , 1–10 (2018).
Google Scholar
Goto, Kensuke, Balachandran Kumarendran, Sachith Mettananda, Deepa Gunasekara, Yoshito Fujii, and Satoshi Kaneko. Analysis of effects of meteorological factors on dengue incidence in Sri Lanka using time series data. PloS one 8 (5) . : e63717 (2013).
Ramírez-Soto M.C., Machuca J.V.B., Stalder D.H., Champin D., Mártinez-Fernández M.G., Schaerer C.E., SIR-SI model with a Gaussian transmission rate: Understanding the dynamics of dengue outbreaks in Lima, Peru. PLoS ONE 18 (4) : e0284263. (2023). 10.1371/journal.pone.0284263
Derouich, M., Boutayeb, A. & Twizell, E. A model of dengue fever. Biomedical engineering online 2 (1), 1–10 (2003).
Lee, C. et al. Epidemic prediction of dengue fever based on vector compartment model and Markov chain Monte Carlo method. BMC bioinformatics 22 , 1–11 (2021).
Reiner, R. C. Jr. et al. A systematic review of mathematical models of mosquito-borne pathogen transmission: 1970–2010. Journal of The Royal Society Interface 10 (81), 20120921 (2013).
Martheswaran, T. K., Hamdi, H., Al-Barty, A., Zaid, A. A. & Das, B. Prediction of dengue fever outbreaks using climate variability and markov chain monte carlo techniques in a stochastic susceptible-infected-removed model. Scientific Reports 12 (1), 5459 (2022).
Article ADS PubMed PubMed Central Google Scholar
Pandey, A., Mubayi, A. & Medlock, J. Comparing vector–host and SIR models for dengue transmission. Mathematical Biosciences 246 (2), 252–259. https://doi.org/10.1016/j.mbs.2013.10.007 (2013).
Article MathSciNet PubMed Google Scholar
Erandi, K. K. W. H., Perera, S. S. N. & Mahasinghe, A. C. Analysis and forecast of dengue incidence in urban Colombo, Sri Lanka. Theoretical Biology and Medical Modelling 18 , 1–19 (2021).
Colombo Municipal Council, https://www.colombo.mc.gov.lk/colombo.php , accessed: 18 April 2023.
Ministry of Transport and Civil Aviation, Sri Lanka, Urban transport system development project for Colombo metropolitan region and suburbs (2008).
Karunathilaka K., Dabare H., Nandalal K., Changes in rainfall in Sri Lanka during 1966-2015. Engineer: Journal of the Institution of Engineers, Sri Lanka 50 (2), 10.4038/engineer.v50i2.7251 (2017).
Dalpadado, R., Amarasinghe. D., Gunathilaka. N., and Ariyarathna. N., Bionomic aspects of dengue vectors Aedes aegypti and Aedes albopictus at domestic settings in urban, suburban and rural areas in Gampaha District, Western Province of Sri Lanka. Parasites & Vectors 15 1-148 (2022).
Herath, J. M. K., Abeyasundara, H. T., De Silva, W. P. P., Weeraratne, T. C., and Karunaratne, S. P., Weather-Based Prediction Models for the Prevalence of Dengue Vectors Aedes aegypti and Ae. albopictus. Journal of Tropical Medicine 1 4494660 (2022).
Noordeen, F. et al. Distribution of dengue vectors, Aedes aegypti and Aedes albopictus, in a few selected semi-urban areas of the Central Province of Sri Lanka. Sri Lankan Journal of Infectious Diseases 8 , 1 (2018).
Santos, C. A. G. et al. Correlation of dengue incidence and rainfall occurrence using wavelet transform for João Pessoa city. Science of The Total Environment 647 , 794–805. https://doi.org/10.1016/j.scitotenv.2018.08.019 (2019).
Thai K. T., Cazelles B., Nguyen N. V., Vo L. T., Boni M. F., Farrar J., Simmons C. P., R. van Doorn H., de Vries P. J., Dengue dynamics in Binh Thuan province, southern vietnam: periodicity, synchronicity and climate variability. PLoS neglected tropical diseases 4 (7) e747. 10.1371/journal.pntd.0000747 (2010).
NASA POWER Data Access Viewer, https://power.larc.nasa.gov/data-access-viewer/ , accessed: January 2023.
Newton, E. A. & Reiter, P. A model of the transmission of dengue fever with an evaluation of the impact of ultra-low volume (ULV) insecticide applications on dengue epidemics. The American journal of tropical medicine and hygiene 47 (6), 709–720 (1992).
Article PubMed Google Scholar
Vector Disease Control International, Mosquito biology 101: Life cycle, https://www.vdci.net/mosquito-biology-101-life-cycle/#: \(\sim\) :text=Females%20with%20an%20adequate%20food,in%20addition%20to%20plant%20nectar. Accessed: 02-01-2024.
Haario, H., Laine, M., Mira, A. & Saksman, E. Dram: Efficient adaptive mcmc. Statistics and computing 16 , 339–354. https://doi.org/10.1007/s11222-006-9438-0 (2006).
Article MathSciNet Google Scholar
The MathWorks, Inc 2024a, https://www.mathworks.com/products/matlab.html
Download references
Acknowledgements
Dengue data for this study were kindly provided by Dr. Sudath Samaraweera, the Director of the National Dengue Control Unit, Ministry of Health of Sri Lanka.
Author information
Authors and affiliations.
Research & Development Centre for Mathematical Modeling, Department of Mathematics, Faculty of Science, University of Colombo, Colombo, 00030, Sri Lanka
Piyumi Chathurangika, S. S. N. Perera & S. A. Kushani De Silva
You can also search for this author in PubMed Google Scholar
Contributions
P. C. - Methodology, Investigation, Simulations, Writing the original draft and editing. S. S. N. P. - Review and discussions. K. D. S - Conceptualize, Investigation, Methodology, Writing the original draft and editing. All authors read and approved the final manuscript.
Corresponding author
Correspondence to S. A. Kushani De Silva .
Ethics declarations
Competing interests.
The authors declare no competing interests.
Additional information
Publisher’s note.
Springer Nature remains neutral with regard to jurisdictional claims in published maps and institutional affiliations.
Supplementary Information
Supplementary information., rights and permissions.
Open Access This article is licensed under a Creative Commons Attribution-NonCommercial-NoDerivatives 4.0 International License, which permits any non-commercial use, sharing, distribution and reproduction in any medium or format, as long as you give appropriate credit to the original author(s) and the source, provide a link to the Creative Commons licence, and indicate if you modified the licensed material. You do not have permission under this licence to share adapted material derived from this article or parts of it. The images or other third party material in this article are included in the article’s Creative Commons licence, unless indicated otherwise in a credit line to the material. If material is not included in the article’s Creative Commons licence and your intended use is not permitted by statutory regulation or exceeds the permitted use, you will need to obtain permission directly from the copyright holder. To view a copy of this licence, visit http://creativecommons.org/licenses/by-nc-nd/4.0/ .
Reprints and permissions
About this article
Cite this article.
Chathurangika, P., Perera, S.S.N. & De Silva, S.A.K. Estimating dynamics of dengue disease in Colombo district of Sri Lanka with environmental impact by quantifying the per-capita vector density. Sci Rep 14 , 24629 (2024). https://doi.org/10.1038/s41598-024-76176-5
Download citation
Received : 24 March 2024
Accepted : 11 October 2024
Published : 20 October 2024
DOI : https://doi.org/10.1038/s41598-024-76176-5
Share this article
Anyone you share the following link with will be able to read this content:
Sorry, a shareable link is not currently available for this article.
Provided by the Springer Nature SharedIt content-sharing initiative
- Differential equations
- Per-capita vector density
- Dengue emergence
- Dengue outbreaks
- Seasonal weather patterns
Quick links
- Explore articles by subject
- Guide to authors
- Editorial policies
Sign up for the Nature Briefing: AI and Robotics newsletter — what matters in AI and robotics research, free to your inbox weekly.

- Research article
- Open access
- Published: 02 June 2010
Air pollution and health in Sri Lanka: a review of epidemiologic studies
- Yatagama Lokuge S Nandasena 1 ,
- Ananda R Wickremasinghe 2 &
- Nalini Sathiakumar 3
BMC Public Health volume 10 , Article number: 300 ( 2010 ) Cite this article
32k Accesses
46 Citations
6 Altmetric
Metrics details
Air pollution is increasingly documented as a threat to public health in most developing countries. Evaluation of current air quality levels, regulatory standards and scientific literature on outdoor and indoor air pollution, and health effects are important to identify the burden, develop and implement interventions and to fill knowledge gaps in Sri Lanka.
PUBMED and Medline databases, local journals and conference proceedings were searched for epidemiologic studies pertaining to air pollution and health effects in Sri Lanka. All the studies pertaining to air pollution and health effects were considered.
Sixteen studies investigated the association between exposure to ambient or indoor air pollution (IAP) and various health outcomes ranging from respiratory symptoms, low birth weight and lung cancers. Of the sixteen, three used a case control design. Half of the studies collected exposure data only through questionnaires. There were positive associations between air pollution and adverse health effects in all studies. Methodological limitations in most of the studies resulted in poor quantification of risk estimates.
A limited number of epidemiological studies in Sri Lanka have investigated the health effects of air pollution. Based on findings of studies and reported air quality levels, air pollution may be considered a neglected public health problem in Sri Lanka.
Peer Review reports
Air pollution, both indoors and outdoors, is a major environmental health problem affecting people in both developed and developing countries. Although air pollutants are many, the most important are particle pollution (often referred to as particulate matter (PM)), ground-level ozone (O 3 ), carbon monoxide (CO), sulfur oxides (SO x ), nitrogen oxides (NO x ), and lead (Pb) which are found in the ambient air (also known as "criteria pollutants"); PM, CO, SO x , NO x , environmental tobacco smoke (ETS), formaldehyde and polycyclic organic matter are found indoors [ 1 , 2 ].
Exposure to air pollutants leads to a variety of health effects depending on the type of pollutant, amount of the pollutant exposed to, duration and frequency of exposure, and associated toxicity of the specific pollutant. These exposures are associated with a broad range of acute and chronic health effects varying from sub-clinical effects to premature mortality [ 3 ]. Although air pollutants are categorized in a number of different ways, most air pollutants generally do not occur in isolation, but in complex mixtures that create the potential for synergistic effects among them [ 4 ]. The composition of air pollutants and their associated toxicity vary in different settings. Age, cultural practices, life style and socio-economic status may influence the exposure to air pollutants [ 4 ]. As the individual sensitivity to pollutants increases, the severity of the response will increase for a given pollutant. Therefore, the effects of air pollutants and the severity of health outcomes in a given population depend on the population sensitivities [ 3 ]. Hence, the health impact of air pollution on a given community cannot be directly generalized from results of studies in other settings.
Several population groups are more vulnerable to the effects of air pollutants; those who are innately susceptible more than others, those who become susceptible as a result of environmental, social and personal behaviours, and those who are simply exposed to unusually large amounts of air pollutants [ 5 ]. Groups that are more sensitive to air pollutants include unborn and young children, elderly people, and those with a history of cardio-respiratory diseases [ 5 ]. Hence, the demographic profile of a given population is also important.
Although air pollution is recognized as an emerging public health problem in developing countries, most of these countries do not have adequate data to evaluate the actual magnitude of the problem. The main reason may be that air pollution co-exists with other important public health problems, such as communicable diseases, vector-borne diseases, malnutrition and poor sanitation, which are given higher priority in circumstances where economic resources are limited. This has delayed the actions needed to adequately assess, evaluate and control air pollution [ 4 ]. The Sri Lankan situation is no different to other developing countries.
Our primary interests were to explore the air quality levels and review the literature regarding health effects due to air pollution in Sri Lanka. In the first part of this review, we discuss air quality, control measures implemented, and air quality regulations in Sri Lanka separately for ambient and indoor air. Secondly, we review the studies pertaining to health effects due to air pollution in Sri Lanka.
Ambient air pollution
Ambient air pollution, especially in urban environments, arises from a spectrum of different sources, which are broadly classified as stationary, mobile, and area emission sources. The main source of ambient air pollution in Sri Lanka is vehicular emissions, which contributes to over 60% of total emissions in Colombo [ 6 ].
Measures have been taken to reduce outdoor air pollution due to vehicular emissions. The National Policy on Urban Air Quality Management was adopted in 2000. The phasing out of leaded gasoline in June 2002, introduction of low sulphur diesel in January 2003, banning the importation of Two Stroke Three-wheelers in 2008, and initiation of vehicular emission testing programme in year 2008 are some key steps that have been taken to control urban outdoor air pollution in Sri Lanka. The permissible ambient air quality standards for selected air pollutants were for the first time enacted under the National Environmental (Ambient Air Quality) Regulations of 1994 [ 7 ]. With the publication of WHO air quality guidelines in 2005[ 8 ], air quality standards for Sri Lanka, including standards for PM 10 and PM 2.5, were amended and gazetted in August 2008[ 9 ] (Table 1 ).
Despite many discussions to expand air quality monitoring in Sri Lanka, there has been only one station located at Colombo Fort, since 1997, to monitor ambient air quality on a continuous basis. Based on data from this station, the average annual ambient PM 10 levels in Colombo over the years have remained relatively stable ranging from 72 to 82 μg/m 3 (Figure 1 ). The World Health Organization (WHO) recommends that the average annual ambient PM 10 level be <20 μg/m 3 [ 8 ]. Comparison of coarse particle mass concentrations of the Asian region under the Regional Co-operation Agreement (RCA) shows that the three-year average values reported from Colombo Fort (an urban location) was 73.37 μg/m 3 and in a residential area was 58.82 μg/m 3 from 2002 to 2005. Special 24-hour measurements for the purpose of the inter-country comparison were carried out at the Colombo Fort monitoring station on a weekly basis using the Gent stacked filter unit particle samplers. The values reported from other regional locations (used the same methodology and instruments) were: Bangladesh urban location - 45.76 μg/m 3 ; India-Trombay - 37.34 μg/m 3 ; India-Vashi - 82.83 μg/m 3 ; Pakistan - 67.45 μg/m 3 , Thailand, urban - 38.67 μg/m 3 , Thailand, suburban - 25.77 μg/m 3 and Vietnam - 50.29 μg/m 3 [ 10 ]. Another comparison of several cities around the world reported that the PM 10 and SO 2 levels in Colombo (based on the Colombo Fort monitoring station) is unhealthier than cities such as Hong Kong, Singapore, Bangkok Taipei and Tokyo, and the PM 10 levels in Colombo are similar to that of Ho Chi Minh, Jakarta and Mumbai [ 11 ]. Hourly averages of SO 2 have exceeded the Sri Lankan standards on 177 occasions from May 2003 to December 2006. The annual mean levels of SO 2 have not exceeded the USEPA limit of 80 μg/m 3 , but the levels have shown an increasing trend in the last few years (Figure 2 ). NO 2 levels in the Colombo city over the past few years have shown an increasing trend although the levels did not exceed the Sri Lankan standards (Figure 3 ). High levels of air pollutants were recorded during the North East monsoon period which lasts from mid November to January over the years [ 12 ]. CO and O 3 levels were relatively low in Colombo as compared to other air pollutants [ 13 ]. Many criticisms have been expressed with reference to the Colombo Fort monitoring station underestimating air quality in the Colombo city area. Some of the criticisms include the location of the monitoring station being not more than 800 m from the sea, which causes the shedding off of pollutants due to wind, shifting of high traffic towards the interior of the country due to prevailing high security zone in its vicinity, and newly established factories and power plants being situated several kilometres away from the monitoring station.
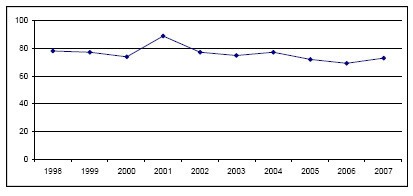
Annual averages of PM-10 at Colombo Fort ambient air quality monitoring Station (1998-2007) . Source: Central Environmental Authority (Year 2007).
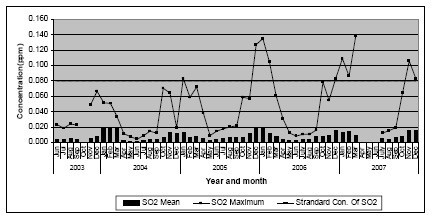
Monthly mean sulfur dioxide concentrations at Colombo Fort (June 2003 - December 2007) . Source: Central Environmental Authority (2007).
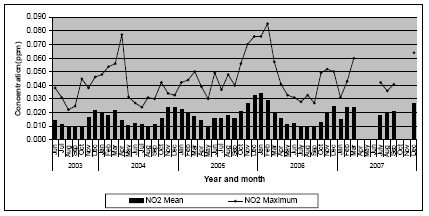
Monthly mean of one-hour averages of nitrogen dioxide concentrations at Colombo Fort (June 2003 - December 2007) . Source: Central Environmental Authority (Year 2007).
The National Building Research Organization of Sri Lanka established a passive air quality monitoring network in 2001 covering 15 locations in the Colombo city to monitor SO 2 and NO 2 [ 14 ]. Mallikarachchi et al [ 15 ], and Manawadu and Wijesekara [ 16 ] showed that the air quality of Colombo city was significantly associated with land transport density, population and building density by modelling air quality in the Colombo city, using data from the passive sampling air quality monitoring network.
Indoor air pollution
Indoor exposures are a result of complex interactions between the structure, building systems, indoor source strength, removal and deposition rate within the structure, indoor mixing and chemical reactions, furnishings, the outdoor environment, and the practices and the behaviours of the inhabitants [ 17 ]. High pollutant concentrations can result inside a closed indoor environment even with relatively modest emissions. When the windows are open and the wind speed is moderate or high, the indoor concentrations of air pollutants may be the same as that outdoors [ 18 ]. Although Sri Lanka being a tropical country is expected to have good indoor ventilation, limited data suggest that indoor air is more polluted than outdoor air [ 19 , 20 ].
Cooking fuel is the main source of IAP in households [ 21 ] while other sources include tobacco smoke and smoke from other outdoor sources [ 1 ]. The types of fuel typically increase in cleanliness, convenience, efficacy and cost as people move up the "energy ladder" [ 1 ]. According to the Demographic and Health Surveys of Sri Lanka of 2000 and 2007, firewood is the principal type of cooking fuel in 78.3% and 78.5% of the households in Sri Lanka, respectively [ 22 ]. It is unlikely that a higher proportion of Sri Lankans will shift to cleaner fuels in the near future [ 23 ]. Most of the traditional local stoves using firewood have incomplete combustion resulting in high pollutant emissions. This coupled with poor ventilation can produce very high levels of indoor pollution [ 24 ]. A study assessing exposure in kitchens using firewood with traditional stoves reported average PM 2.5 concentrations exceeding 1200 μg/m 3 [ 24 ]. A reduction of IAP was shown by the use of improved wood stoves in various settings in Sri Lanka [ 24 ] although the use of these stoves at the community level is almost negligible. Women and young children who usually stay around their mothers while cooking may be the most vulnerable to IAP due to cooking fuel.
A cross sectional study conducted to measure SO 2 and NO 2 levels indoors and outdoors of 30 low-income households at five different locations in Colombo reported that indoor pollutant levels were higher than those outdoors in all households. In that study, parents maintained a daily activity diary for their 5 to 8 year-old children which showed that these children spent an average 41 hours (out of 48 hours) inside their houses during weekends. The health status of children was not assessed in this study [ 25 ]. Regular use of mosquito coils by 12% of households may be another source of IAP [ 22 ] especially in poorly ventilated houses; the health impact of this exposure has not been investigated in Sri Lanka.
The National Authority on Tobacco and Alcohol (NATA) Act [ 26 ] banned smoking in health-care institutions, educational institutions, government facilities, universities, indoor offices, and other indoor workplaces. The Global Youth Tobacco Survey (GYTS) reported that there is no marked reduction in second-hand smoke in public places following the implementation of the NATA Act [ 27 ]. The GYTS further reported that there is a reduction in the prevalence of parental smoking from 1999 (50.8%, 95%CI = 47.8%-53.8%) to 2007 (29.9%, 95% CI = 25.6%-34.5%). With almost one third of parents currently smoking, second-hand smoke in households may still be high and need to be explored quantitatively.
Search strategy
We searched literature from PUBMED and MEDLINE databases to identify all relevant publications prior to June 2009. The following search terms were used: "ambient air pollution AND Sri Lanka", "indoor air pollution AND Sri Lanka", "air pollution AND Sri Lanka", "epidemiologic studies AND Sri Lanka", "health effects AND Sri Lanka."
We manually identified additional studies published in local journals and included conference proceedings which were inaccessible via the electronic databases. Reference lists of original studies were checked for related studies (cross reference). Authors of selected studies were contacted to identify additional literature.
Study selection
We included all studies relating to health effects due to air pollution in Sri Lanka irrespective of the study design. Studies that actually measured air quality levels and studies using proxy variables to predict air quality, indoors, outdoors or both, were included. We excluded studies which did not have health outcomes, and studies presented as conference proceedings which were not supplemented with a full report. We considered only the studies published in English as there are no scientific journals or any scientific literature available in the local languages. Preliminary results of ongoing studies were not considered.
Data extraction
Two investigators reviewed each paper independently. Discrepancies were discussed and agreement was achieved with consensus. Papers were categorised as indoor or outdoor based on the methodology. Studies were further categorised according to the study design. The following information from each study was abstracted: study location, exposure assessment, health outcomes, other risk factors evaluated, study results and limitations.
Results and Discussion
Study characteristics.
We retrieved 65 publications and conference proceedings of potential interest, of which, 38 were excluded initially as they did not have a health outcome resulting from air pollution. Duplications of studies as publications and conference proceedings were taken as a single study. We finally identified 17 original studies. One study was excluded as we were unable to find the full report of the abstract presented in the conference proceeding (Figure 4 ). Studies included are summarized in Tables 2 & 3 . Ten and six studies focussed on outdoor air pollution (Table 2 ) and indoor air pollution (Table 3 ), respectively. Nine studies had used a cross-sectional study design. Most of the health outcome studies show positive associations between air pollution and various adverse health effects, which correspond to results from studies conducted in other countries [ 1 , 5 ]. However, some studies have methodological limitations due to lack of objective measurement of personal exposures and indoor air quality leading to potential misclassification. Apart from age and sex, most studies do not adjust for potential confounding factors such as sources of air pollution other than those described in the study. Furthermore, possible factors which may influence indoor air pollution levels such as ventilation of houses, location of the kitchen, stove types and availability of exhaust mechanisms to expel the generated smoke from biomass fuel combustion, smoking inside households and mosquito coil use were poorly quantified (Figure 4 ).
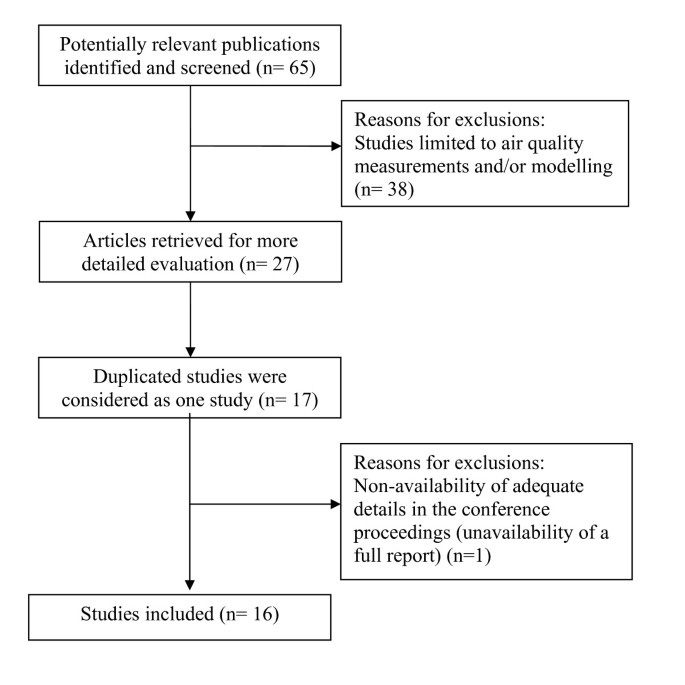
Flow diagram of selection of studies .
Studies on Ambient Air Pollution
We identified 10 studies (Table 2 ) that examined the effect of ambient air pollution exposure on various respiratory health outcomes. Of the 10, 7 studies adopted a cross-sectional design. In all studies, questionnaires and/or passive/active samplers were used to ascertain exposure. None of the studies used personal monitoring of exposure to ambient air. Other methodological limitations include small study sizes, potential misclassification of exposure, recall biases and inadequate adjustment for potential confounders.
Respiratory health
Sirithunga et al.[ 28 ] measured outdoor air pollutant levels in home environments of school children in an urban and a rural area in the Kandy district and assessed the respiratory symptoms over a one-year period on a daily basis using health diaries. Outdoor measurements representing a large area were obtained with passive samplers and indoor exposure relied on proxy parameters. The authors minimized recall bias by collecting information on the health conditions on a daily basis. The occurrence of cough, nasal discharge and throat irritation were 1.12 (95%CI = 1.05-1.19), 1.17 (95%CI = 1.09-1.24) and 1.48 (95%CI = 1.31-1.67) times higher among children in the Kandy urban area, respectively, as compared to those of the rural area. Lung functions were also measured but there were no significant differences in the measurements between urban and rural subjects. The major limitation was the potential for exposure misclassification.
Premarathna et al. [ 29 ] studied the effects of air pollution on health of residents in an industrial area in Sri Lanka using a cross-sectional design and reported that the adult population living in the industrial area was 2.1 times more likely to have unexplained episodic cough (95% CI = 1.13-7.09) and a significant reduction in expiratory flow as compared to the adult population living in a non-industrial area. The younger population (≤ 12 years) living in the industrial area were 2.8 (95% CI = 1.10-7.10) times more likely to have rhinitis as compared to the younger population living in the non-industrial area. These conclusions were based on the assumption that the industrialized area is more polluted compared to the control area. No air quality measurements were made to confirm this assumption.
School children are another group exposed to high levels of pollutants exaggerated by overcrowding of major schools in cities, especially in Colombo. The prevalence of respiratory symptoms among school children attending a school in Colombo situated close to a busy main road was significantly higher than that of children attending a school situated in a rural area (cough - OR = 1.33, 95% CI = 1.12-2.48; presence of phlegm - OR: 1.66, 95% CI = 1.12-2.46; wheezing - OR: 1.29, 95% CI = 0.80- 2.10). Children's exposure in the home environment was considered only by proxy parameters such as cooking fuel type and no adjustments were made for confounding factors. Air pollutant (NO 2 , SO 2, and TSP) levels were significantly higher in the premises of the urban school as compared to the rural school [ 30 ]. The respiratory health of school children (12 to 16 years), residing in and around Kandy city (n = 770), was studied by measuring PM 10 levels in a cross-sectional survey[ 31 ]. PM 10 levels "outside-the-city school" were lower (3-hour average, 84 mg/m 3 ) as compared to the "city school" (121 mg/m 3 ). An arbitrary health indicator of 1, a measure of perfect health, was found among 32% of children of the "outside-the-city school" as compared to only 8% of children of the "city school". Misclassification of exposure is a potential limitation of the study as the total time spent at the school by a secondary school student is less than 15% for a one-year life span and the exposure levels in other living environments of the children were not considered. Further, considerable proportions of children in urban schools travel daily from rural locations with low outdoor exposure. Children from rural households may be exposed to more IAP due to more biomass fuel use in these areas.
Mistry R. et al. [ 32 ] compared the prevalence of asthma among 13 to 14 year-old children in Galle (n = 1162) (described by the authors as an unplanned, busy city with predominant use of kerosene or firewood for cooking in Sri Lanka) with Chandigarh (n = 575) (a city defined by the authors as a planned, clean and using smoke-free household fuel in India). Children in Galle were at an increased risk for life-time wheezing (Galle-28.7%, Chandigarh-12.5%; the prevalence odds ratios (POR) = 2.3, 95% CI = 1.8-2.9) and for wheezing in the previous year (Galle-21.9%, Chandigarh-10.4%, POR = 2.1, 95% CI = 1.6-2.7) as compared to children from Chandigarh.
An ecologic study examined the air pollutant levels measured at the Colombo Fort monitoring station and rates of hospital attendance for wheezing needing emergency treatment at the Lady Ridgeway Hospital for Children in Colombo. About 30,932 children required nebulizer therapy in the emergency treatment unit (median daily attendance, n = 85) during the 12-month period beginning in July 1998. The highest number of episodes of nebulization occurred on the most polluted day (with respect to SO 2 and NO 2 ) and the lowest number of nebulizations occurred on the least polluted day in a given week, in a significant number of weeks throughout the year (binomial test, p = 0.05) [ 33 ]. However, the actual exposure of all the patients admitted to this hospital probably did not represent the air quality of Colombo Fort monitoring station as the hospital is a referral centre for the whole country. Exposure data was limited to only a few pollutants.
Based on data of the Colombo Fort monitoring station, episodes of bronchitis, emphysema and other chronic obstructive pulmonary diseases had a strong association with PM 10 levels (correlation coefficient = 0.717; p = 0.01). Nearly 20% of asthma patients who visited the Lady Ridgeway Hospital for Children in Colombo in 2005 could be attributed to exposure to PM 10 in Colombo based on the health impact assessment software developed by WHO [ 34 ]. Although the location of the Colombo Fort monitoring station may not represent the entire area of study population, this study provides evidence for implementation of early mitigation strategies.
Air pollutants were measured using passive samplers in Mount Lavinia, a metropolitan area bordering Colombo, to measure the 24-hour average levels among city dwellers engaged in different occupations[ 35 ]. Bus drivers were exposed to more NO 2 (57.36 μg/m 3 ) and SO 2 (82.70 μg/m 3 ) as compared to trishaw drivers (NO 2 - 50.18 μg/m 3 ; SO 2 - 78.36 μg/m 3 ), shop keepers (NO 2 - 54.91 μg/m 3 ; SO 2 - 63.29 μg/m 3 ) and outdoor vendors (NO 2 - 37.66 μg/m 3 ; SO 2 - 35.25 μg/m 3 ). Respiratory conditions were assessed in each participant using a questionnaire. The highest prevalence of respiratory symptoms was reported among bus drivers.
Blood lead levels
Following the introduction of unleaded gasoline, atmospheric lead levels reduced by 81.5%, 82% and 84% in three locations in Colombo [ 36 ]. Senanayake et al. [ 37 ] measured blood lead levels of a sample of children living near a traffic congested junction in Colombo in 1998 and then one year after the introduction of unleaded gasoline. In 1998, 6% of children had blood lead levels above 10 μg/dL; in 2003, not a single child had a blood lead level >10 μg/dl (range 1.67 μg/dl to 9.7 μg/dl).
Amarasinghe et al. [ 38 ] measured blood lead levels of traffic policemen (n = 64) and non traffic policemen (n = 64) based in Colombo. The mean blood lead levels in traffic and non-traffic policemen were 7.47 μg/dl (SD = 2.89) and 7.06 μg/dl (SD = 2.93), respectively. Abdominal discomfort, tremor and hypertension were higher in traffic policemen as compared to non-traffic policemen, although the differences were not significant. There may be exposure misclassification as non-traffic policemen are duty bound to control traffic during busy hours.
Studies on Indoor Air Pollution
We reviewed 6 studies that have evaluated IAP and health outcomes in Sri Lanka. Of the 6 studies, 3 were case-control studies and 3 were cross-sectional studies. Five of the 6 studies used proxy measurements for IAP and no quantitative measurements were undertaken. There was no adjustment done for potential confounding factors.
Respiratory Health
A hospital-based case-control study [ 39 ] of 300 subjects (age-matched) found that the presence of dust at home was a significant risk factor (adjusted OR = 1.3, 95% CI = 1.02 - 2.1) for asthma. There may have been potential exposure misclassification and recall bias with cases over-reporting a "dusty environment".
A cross-sectional study conducted in a Colombo suburb reported that the use of fire wood for cooking was a significant risk factor for respiratory symptoms (OR = 1.61; 95% CI = 1.3 - 2.53) [ 20 ]. Average TSP levels in houses using firewood were 0.606 mg/m 3 and in houses not using firewood were 0.245 mg/m 3 . The respirable dust concentration exceeded the WHO standards in 84% of households using firewood and 54% of the households not using firewood [ 20 ].
Another school-based cross-sectional study of asthmatics (n = 441) and non- asthmatics (n = 1510) in the Gampaha district reported that the presence of firewood smoke in the bed room while cooking (OR 1.4, 95% CI 1.1-1.9), use of mosquito coils (OR 1.5, 95% CI 1.2 - 1.9), and a dusty environment (OR 1.8, 95% CI 1.4 - 2.3) significantly increased the risk of asthma. No air pollutant measurements were done. The environmental exposure assessment was based on a questionnaire and no adjustment for confounding factors was done [ 40 ].
Ranasinghe et al. [ 41 ] reported an association (p < 0.05) between biomass exposure and cataract by comparing cataract patients (cases, n = 197) with patients admitted to the National Eye Hospital, Colombo, for other eye problems (controls, n = 190). The biomass exposure assessment was solely based on a questionnaire.
Lung Cancer
Perera et al. [ 42 ] investigated the association between exposure to biomass smoke and lung cancer. The majority of the population (more than 50%) was between the ages of 41-50 years and male; the majority of the cases were directly exposed to tobacco smoke (48%). There was an association between smoking and lung cancer (p = 0.04) but not with biomass fuels use (p = 0.10). Exposure was assessed using a questionnaire.
Low Birth Weight
A cross-sectional study [ 43 ] reported that the availability of a separate kitchen (OR = 2.7, 95% CI = 1.6-4.7), using less clean cooking fuel (OR = 3.9, 95% CI =1.8 - 8.5) and not having adequate ventilation in the cooking area (OR = 2.7, 95% CI =1.3 - 5.3) were significant predictors of low birth weight in the Kegalle and Kalutara districts. Air quality measurements were not reported in this study.
Confounders
Several studies[ 28 , 30 , 35 , 39 , 40 ] adjusted for common confounders such as second-hand smoke exposure, cooking fuel type, mosquito coil use etc., proxy measures for indoor air quality. The air quality levels in studies that measured air quality [ 20 , 30 , 34 , 35 ] were similar to those reported in other countries.
Strengths and Weaknesses
We did not use strict inclusion criteria as our primary objective was to explore most of the studies done in Sri Lanka relevant to the subject. We included studies that used only proxy variables as the number of studies that measured actual air quality was limited. We had no option but to include as many studies as possible due to the limited number.
Future Perspectives
The broad range of likely health impacts of air pollution in Sri Lanka means that a variety of methods and strategies should be applied for mitigating adverse health effects and minimizing exposures. Generating baseline data related to indoor and outdoor air pollutants and human health will form the platform to address this problem at national, community and individual level. It will be the basis for advocacy, formulating mitigation strategies and minimizing exposure.
The lack of a proper air quality monitoring system to track human exposure is a major limitation. This has to be addressed to determine the impact of programmes and to identify the future directions. The availability of stringent standards by itself is of no use if the air quality that citizens are exposed to is unknown to identify areas for intervention using an evidence based approach. Therefore, establishing a modern ambient air quality monitoring network, at least covering the main busy cities in the country, is an early need.
Although several activities have been implemented to reduce outdoor air pollution, there are no specific interventions implemented at national level to reduce IAP or to minimize the exposure of vulnerable groups to indoor air pollutants. There is a lack of reliable indoor air quality data and determinants of indoor air quality in Sri Lanka, a priority that needs to be addressed when estimating the burden of disease associated with IAP. Enactment of new laws and enforcing existing laws will require reliable baseline data on indoor and outdoor air quality and health impact. Practices and other determinants that increase human exposure to air pollutants need to be identified in local communities. Robust research studies should be designed to generate individual exposure data, identify and evaluate determinants associated with air pollution exposures and to quantify the public health effects of such exposures in Sri Lanka. Public health impact of outdoor air pollution control activities should be assessed to monitor and modify such mitigation activities.
Modifying existing regulatory practices based on findings of robust research studies, strict adherence to regulations at community and household level and identifying new mitigation strategies can play a key role in minimizing the impact of air pollution on health.
Conclusions
Despite inconsistencies, findings from Sri Lankan studies generally suggest that air pollution, both ambient and indoor, is a major public health problem in Sri Lanka. The results of these studies could be used as a base to design larger epidemiologic investigations to address the problems of measurement error, reduce uncertainties in risk estimates and identify the determinants of exposures.
Bruce N, Perez-Padilla R, Albalak R: The health effects of indoor air pollution exposure in developing countries. 2002, Geneva: World Health Organization, 11-
Google Scholar
Senevirathne SRDA: Air pollution: a case study of environmental pollution. Journal of College of Community Physicians of Sri Lanka. 2003, 8 (1): 1-9.
American Thoracic Society: What Constitutes an Adverse Health Effect of Air Pollution?. American Journal of Respiratory Critical Care Medicine. 2000, 161: 665-673.
Article Google Scholar
HEI: Special Report 15, Health Effects of Outdoor Air Pollution in Developing Countries of Asia: A literature Review. 2004, Health Effects Institute
WHO: Health Aspects of Air Pollution: Results from the WHO project " Systematic Review of Health Aspects of Air Pollution in Europe". 2004, Europe: World Health Organization
Batagoda B, Sugathapala A, Yalegama M, Jayasinghe B: Urban Air Quality Management in Sri Lanka. 2004, Colombo: Air Resource Management Center (AirMAC), Ministry of Environment and Natural Resources, Sri Lanka, iii-
The National Environmental Act, No. 47 of 1980: Amendment to the National Environmental (Ambient Air Quality) Regulations, No 817/6-Tuesday, May 03, 1994. Volume No 817/6-Tuesday, May 03, 1994. 1994, Government Notification: The Gazette of the Democratic Socialist Republic of Sri Lanka
WHO: WHO Air quality guidelines for particulate matter, ozone, nitrogen dioxide and sulfur dioxide, Global update 2005, Summary of risk assessment. 2005, Geneva: World Health Organization
The National Environmental Act, No. 47 of 1980: Amendment to the National Environmental (Ambient Air Quality) Regulations, No 1562/22-Friday, August 15,2008. 2008, Government Notification: The Gazette of the Democratic Socialist Republic of Sri Lanka
Hopke PK, Cohen DD, Begum BA, Biswas SK, Ni B, Pandit GG, Santoso M, Chung YS, Davy P, Markwitz A, et al: Urban air quality in the Asian region. Sci Total Environ. 2008, 404 (1): 103-112. 10.1016/j.scitotenv.2008.05.039.
Article CAS PubMed Google Scholar
Air Quality in Asian Cities. [ http://www.cleanairnet.org/caiasia/1412/articles-59689_AIR.pdf ]
Jayaratna RNR, Ileperuma OA: Air Quality Trends and Variation Pattern of Air Pollutants in the City of Colombo. "Air That We Breath", Third National Symposium on air resource management in Sri Lanka: 2007; Colombo. 2007
Jayawardana KGS, Jayaratna RNR: What Colombo's Air Quality Tell Us. "Air That We Breath", First National Symposium on Air Resource Management in Sri Lanka 2004; Colombo. 2004
Samarakkody RP, Premasiri HDS, Annakkage CJ, Basnayake GBMA: Identification of Critical and Shrinking Areas in Colombo by Means of Spatial Air Quality Monitoring. "Air That We Breathe", First National Symposium on Air Resource Management in Sri Lanka: 2004; Colombo. 2004
Mallikarachchi BS, Manawadu L, Samarakkody RP: Identification of Air Pollution Potential Areas and Its Temporal and Partial Pattern of the City of Colombo - Applying GIS. "Air That We Breath", First National Symposium on Air Resource Management in Sri Lanka 2004: 2004; Colombo. 2004
Manawadu L, Wijesekara KASS: Understanding Air Pollution Dynamics and Exploring Reasons for Aggravation of Air Pollution in the City of Colombo Using Geo-Statistical Techniques. "Geo-Informatics For National Development", The Sixth National Symposium on Geo-Informatics: 2009; Colombo. 2009, 17-32.
Mitchell CS, Zhang J, Sigsgaard T, Jantunen M, Lioy PJ, Samson R, Karol MH: Current State of the Science: Health Effects and Indoor Environmental Quality. Environmental Health Perspectives. 2007, 115 (6): 958-964. 10.1289/ehp.8987.
Article PubMed PubMed Central Google Scholar
WHO: Monitoring ambient air quality for health impact assessment. European Series. 1999, Copenhagen: Regional Office for Europe, 9-14,19
Samarakkody RP, Fernando ATR: Measuring of Nitrogen Dioxide Exposure Levels - A Quick Test for Air Pollution. "Air That We Breath", Third National Symposium on air resource management in Sri Lanka: 2007; Colombo. 2007
Lankathilaka KN, Seneviratne SRDA, Fernando DN: Indoor air quality and respiratory symptoms among children and women. Sri Lanka Association for the Advancement of Science - 56th Annual Session. Volume Part - 1 abstract. Colombo. 2000
Bruce N, Perez-Padilla R, Albalak R: Indoor air pollution in developing countries: a major environmental and public health challenge. Bulletin of the World Health Organization. 2000, 78 (9): 1078-1092.
CAS PubMed PubMed Central Google Scholar
Demographic and Health Survey 2006/2007, Preliminary Report. [ http://www.statistics.gov.lk ]
Nandasena YLS, Wickremasinghe AR, Sathiakumar N: Socio-demographic characteristics and principal cooking fuel type in Sri Lanka: Comparison of data from two Demographic and Health surveys. 14th Annual Academic Sessions of College of Community Physicians of Sri Lanka: 2009; Colombo. 2009
Amerasekera RM: Proven interventions to reduce indoor air pollution due to cooking with biomass. "Air That We Breath", First National Symposium on Air Resource Management in Sri Lanka: 2004; Colombo. 2004
Senanayaka MP, Samarakkody RP: Impact of outdoor and Indoor air pollution on personal exposure of 5 - 8 year old children living in low income settlements in Colombo. "Air That We Breathe", First National Symposium on Air Resource Management in Sri Lanka. Colombo. 2004
National authority on tobacco and alcohol act, no. 27. [ http://www.documents.gov.lk/actspg/acts2006-2.htm ]
Gunasekara P, Rahman K, Warren C, Lee J, Lea V, Asma S: Tobacco use among students aged 13-15 years--Sri Lanka, 1999-2007. MMWR Morbidity and Mortality Weekly Report. 2008, 57 (20): 545-549.
Sirithunga TLJC, Kumarasiri RPK, Illeperuma OA: Effects of outdoor air pollution on the respiratory health of children in a rural and an urban area in the Kandy district. "Air That We Breath", Second National Symposium on Air Resource Management in Sri Lanka: 2006; Colombo. 2006
Premaratna R, Pathmeswaran A, Chandrasekara B, Dissanayake AS, Silva HJD: Effects of Pollution on Health of Residents in an Industrial Area in Sri Lanka. Archives of Environmental Health. 2002, 57 (6): 579-583. 10.1080/00039890209602091.
Nandasena YLS, Perera GBS, Emmanuel R, Premasiri HDS: Exposure to aerosol pollution and reported respiratory symptoms among school children in segments of urban and rural settings in Sri Lanka. "Air That We Breath", Third National Symposium on air resource management in Sri Lanka: 2007; Colombo, Sri Lanka. 2007
Elangasinghe MA, Shanthini R: Traffic Related Air Pollution And Respiratory Related Health of Students Attending School in Kandy. "Air That We Breath", Second National Symposium on Air Resource Management in Sri Lanka: 2006; Colombo. 2006
Mistry R, Wickramasingha N, Ogston S, Singh M, Devasiri V, Mukhopadhyay S: Wheeze and urban variation in South Asia. Eur J Pediatr. 2004, 163 (3): 145-147. 10.1007/s00431-003-1393-6.
Article PubMed Google Scholar
Senanayake MP, Samarakkody RP, Sumanasena SP, Kudalugodaarachchi J, Jayasinhe SR, Hettiarachchi AP: A relational analysis of acute wheezing and air pollution. Sri Lanka Journal of Child Health. 2001, 30 (2): 66-68.
Thishan Dharshana KG, Coowanitwong N: Ambient PM(10) and respiratory illnesses in Colombo City, Sri Lanka. J Environ Sci Health A Tox Hazard Subst Environ Eng. 2008, 43 (9): 1064-1070.
Perera GBS, Emmanuel R, Nandasena YLS, Premasiri HDS: Exposure to aerosol pollution and reported respiratory symptoms among segments of urban and rural population in Sri Lanka. Built - Environment Sri Lanka. 2007, 7 (2): 31-39.
NBRO: Changing atmospheric lead levels in Colombo with discontinuation of leaded petrol in Sri Lanka. Division of Environment. 2003, Colombo: NBRO
Senanayake MP, Rodrigo MDA, Malkanthi R: Blood lead levels of children before and after introduction of unleaded petrol. Ceylon Medical Journal. 2004, 49 (2): 60-61.
PubMed Google Scholar
Amarasinghe JNP: Blood lead levels of traffic policeman in the city of Colombo. 2002, University of Colombo, Postgraduate Institute of Colombo
Karunasekara KAW, Jayasinhe JA, Alwis LW: Risk factors of childhood asthma: a Sri Lankan study. Journal of Tropical Pediatrics. 2001, 47 (3): 142-145. 10.1093/tropej/47.3.142.
Karunasekara KAW, Perera KPJ, Perera MTPR, Abeynarayana J: Genetic and environmental risk for asthma in children aged 5-11 years. Sri Lanka Journal of Child Health. 2005, 34: 79-83.
Ranasinghe MH, Mahanama KRR: Risk of Cataract Formation with Exposure to Biomass Smoke. "Air That We Breath", First National Symposium on Air Resource Management in Sri Lanka: 2004; Colombo. 2004
Perera MAKKP, Mahanama KRR: Investigation of Lung Cancer to Human Activities in Sri Lanka. "Air That We Breath", First National Symposium on Air Resource Management in Sri Lanka: 2004; Colombo. 2004
Pathirane SM, Mahanama KRR: Low Birth Weights of Infants and Exposure to Smoke from Biomass Fuel during Pregnancy. "Air That We Breath", Second National Symposium on Air Resource Management in Sri Lanka 2006: 2006; Colombo. 2006
Pre-publication history
The pre-publication history for this paper can be accessed here: http://www.biomedcentral.com/1471-2458/10/300/prepub
Download references
Acknowledgements
We acknowledge the support of the University of Alabama at Birmingham (UAB) International Training and Research in Environmental and Occupational Health (ITREOH) program, Grant Number 5D43TW05750 from the Fogarty International Center of the National Institutes of Health and to NASA. The content is solely the responsibility of the authors and does not necessarily represent the official views of UAB, Fogarty International Center, or the National Institutes of Health. Authors thank the Central Environmental Authority of Sri Lanka for providing necessary information.
Author information
Authors and affiliations.
Evaluation and Research Unit, National Institute of Health Sciences, Ministry of Health, Kalutara, Sri Lanka
Yatagama Lokuge S Nandasena
Department of Public Health, Faculty of Medicine, University of Kelaniya, Kelaniya, Sri Lanka
Ananda R Wickremasinghe
Department of Epidemiology, School of Public Health, University of Alabama at Birmingham, Birmingham, USA
Nalini Sathiakumar
You can also search for this author in PubMed Google Scholar
Corresponding author
Correspondence to Yatagama Lokuge S Nandasena .
Additional information
Competing interests.
The authors declare that they have no competing interests.
Authors' contributions
YLNS, ARW and NS designed the study. YLSN completed the initial literature survey. YLSN and ARW summarized all eligible papers, synthesized the findings and drafted the manuscript. NS helped in drafting the final manuscript. YLSN is the guarantor of the work. All authors read and approved the final manuscript.
Authors’ original submitted files for images
Below are the links to the authors’ original submitted files for images.
Authors’ original file for figure 1
Authors’ original file for figure 2, authors’ original file for figure 3, authors’ original file for figure 4, rights and permissions.
This article is published under license to BioMed Central Ltd. This is an Open Access article distributed under the terms of the Creative Commons Attribution License ( http://creativecommons.org/licenses/by/2.0 ), which permits unrestricted use, distribution, and reproduction in any medium, provided the original work is properly cited.
Reprints and permissions
About this article
Cite this article.
Nandasena, Y.L., Wickremasinghe, A.R. & Sathiakumar, N. Air pollution and health in Sri Lanka: a review of epidemiologic studies. BMC Public Health 10 , 300 (2010). https://doi.org/10.1186/1471-2458-10-300
Download citation
Received : 12 December 2009
Accepted : 02 June 2010
Published : 02 June 2010
DOI : https://doi.org/10.1186/1471-2458-10-300
Share this article
Anyone you share the following link with will be able to read this content:
Sorry, a shareable link is not currently available for this article.
Provided by the Springer Nature SharedIt content-sharing initiative
- Blood Lead Level
- Global Youth Tobacco Survey
- Mosquito Coil
- Traffic Policeman
- Lady Ridgeway Hospital
BMC Public Health
ISSN: 1471-2458
- General enquiries: [email protected]

COMMENTS
Environmental issues in Sri Lanka include large-scale logging of forests and degradation of mangroves, coral reefs and soil. Air pollution and water pollution are challenges for Sri Lanka since both cause negative health impacts.
Sri Lanka confronted many environmental problems, including water pollution, air pollution, solid waste, deforestation, and biodiversity loss. This paper investigates the environmental issues in...
Sri Lanka confronted many environmental problems, including water pollution, air pollution, solid waste, deforestation, and biodiversity loss. This paper investigates the environmental issues in Sri Lanka and provides insight into the challenges and effects of Environmental Pollution in Sri Lanka.
Increased garbage and environmental pollution are major issues in Sri Lankan urban environments. Moratuwa, Kandy, Colombo, Matale, Gampaha, Negombo are some of the municipalities which are suffering from increased garbage pollution owing to lack of proper dumping or recycling methods.
Grappling with the biggest marine plastic spill in history. The wreck of the X-Press Pearl unleashed a record 1,680 metric tons of plastic pellets on Sri Lanka’s coast in 2021. Scientists want ...
This article examines the environmental dimensions of the current crisis in Sri Lanka, the risks, and the way forward. Sri Lanka, officially declared ‘ bankrupt ’ by its government, is facing the worst crisis in decades.
Impacts of water pollution in Sri Lanka. Keshani Y.H.N. Published 2022. Environmental Science. : Man-made pollution and environmental degradation pose a severe challenge to all Sri Lankans. The main water resource problems in Sri Lanka are due to various human activities such as agriculture, fossil fuel combustion, urbanization, and industrial ...
Nowadays there are lots of environmental issues in Sri Lanka, but most harmful and increasing problems are deforestation, water pollution, and air pollution. So this article explores the environmental issues and their impacts on the people and animals in Sri Lanka.
Dengue is a vector-borne disease transmitted to humans by vectors of genus Aedes causing a global threat to health, social, and economic sectors in many of the tropical countries including Sri Lanka.
Evaluation of current air quality levels, regulatory standards and scientific literature on outdoor and indoor air pollution, and health effects are important to identify the burden, develop and implement interventions and to fill knowledge gaps in Sri Lanka.