Thank you for visiting nature.com. You are using a browser version with limited support for CSS. To obtain the best experience, we recommend you use a more up to date browser (or turn off compatibility mode in Internet Explorer). In the meantime, to ensure continued support, we are displaying the site without styles and JavaScript.
- View all journals
- Explore content
- About the journal
- Publish with us
- Sign up for alerts
- Published: 26 April 2024

Self-assembly of peptide nanocapsules by a solvent concentration gradient
- Haopeng Li ORCID: orcid.org/0009-0008-8945-2180 1 , 2 na1 ,
- Xuliang Qian ORCID: orcid.org/0000-0002-0979-9950 3 na1 ,
- Harini Mohanram 4 ,
- Xiao Han 1 ,
- Huitang Qi 2 ,
- Guijin Zou ORCID: orcid.org/0000-0003-0047-7012 3 , 5 ,
- Fenghou Yuan 2 ,
- Ali Miserez ORCID: orcid.org/0000-0003-0864-8170 1 , 6 ,
- Tian Liu ORCID: orcid.org/0000-0001-9768-5496 2 ,
- Qing Yang ORCID: orcid.org/0000-0001-8623-7532 7 , 8 ,
- Huajian Gao ORCID: orcid.org/0000-0002-8656-846X 3 , 5 , 9 &
- Jing Yu ORCID: orcid.org/0000-0002-4288-951X 1 , 10
Nature Nanotechnology ( 2024 ) Cite this article
2599 Accesses
25 Altmetric
Metrics details
- Drug delivery
- Molecular self-assembly
Biological systems can create materials with intricate structures and specialized functions. In comparison, precise control of structures in human-made materials has been challenging. Here we report on insect cuticle peptides that spontaneously form nanocapsules through a single-step solvent exchange process, where the concentration gradient resulting from the mixing of water and acetone drives the localization and self-assembly of the peptides into hollow nanocapsules. The underlying driving force is found to be the intrinsic affinity of the peptides for a particular solvent concentration, while the diffusion of water and acetone creates a gradient interface that triggers peptide localization and self-assembly. This gradient-mediated self-assembly offers a transformative pathway towards simple generation of drug delivery systems based on peptide nanocapsules.
This is a preview of subscription content, access via your institution
Access options
Access Nature and 54 other Nature Portfolio journals
Get Nature+, our best-value online-access subscription
24,99 € / 30 days
cancel any time
Subscribe to this journal
Receive 12 print issues and online access
251,40 € per year
only 20,95 € per issue
Buy this article
- Purchase on Springer Link
- Instant access to full article PDF
Prices may be subject to local taxes which are calculated during checkout
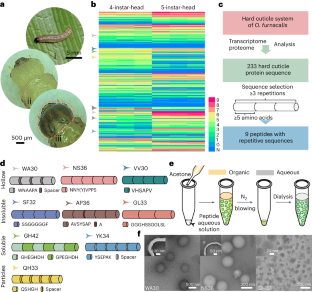
Similar content being viewed by others
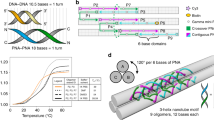
Modular self-assembly of gamma-modified peptide nucleic acids in organic solvent mixtures
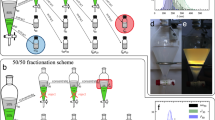
Interrogating helical nanorod self-assembly with fractionated cellulose nanocrystal suspensions
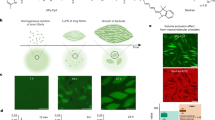
Supramolecular polymers form tactoids through liquid–liquid phase separation
Data availability.
The data that support the plots within this paper and other findings of this study are available via DR-NTU at https://doi.org/10.21979/N9/DJV2BM (ref. 58 ). The data that support the findings of this study are also available from the corresponding authors upon reasonable request. Source data are provided with this paper.
Code availability
All code and scripts used in this study are available from the corresponding authors upon reasonable request.
Bar-Cohen, Y. Biomimetics—using nature to inspire human innovation. Bioinspir. Biomim. 1 , P1 (2006).
Article PubMed Google Scholar
Liu, Z., Meyers, M. A., Zhang, Z. & Ritchie, R. O. Functional gradients and heterogeneities in biological materials: design principles, functions, and bioinspired applications. Prog. Mater. Sci. 88 , 467–498 (2017).
Article CAS Google Scholar
Waite, J. H., Lichtenegger, H. C., Stucky, G. D. & Hansma, P. Exploring molecular and mechanical gradients in structural bioscaffolds. Biochemistry 43 , 7653–7662 (2004).
Article CAS PubMed Google Scholar
Miserez, A., Li, Y., Waite, J. H. & Zok, F. Jumbo squid beaks: inspiration for design of robust organic composites. Acta Biomater. 3 , 139–149 (2007).
Ho, S. P., Marshall, S. J., Ryder, M. I. & Marshall, G. W. The tooth attachment mechanism defined by structure, chemical composition and mechanical properties of collagen fibers in the periodontium. Biomaterials 28 , 5238–5245 (2007).
Article CAS PubMed PubMed Central Google Scholar
Meyers, M. A., McKittrick, J. & Chen, P.-Y. Structural biological materials: critical mechanics–materials connections. Science 339 , 773–779 (2013).
Fratzl, P. & Weinkamer, R. Nature’s hierarchical materials. Prog. Mater. Sci. 52 , 1263–1334 (2007).
Heinemann, F., Launspach, M., Gries, K. & Fritz, M. Gastropod nacre: structure, properties and growth—biological, chemical and physical basics. Biophys. Chem. 153 , 126–153 (2011).
Chen, P.-Y., Stokes, A. & McKittrick, J. Comparison of the structure and mechanical properties of bovine femur bone and antler of the North American elk ( Cervus elaphus canadensis ). Acta Biomater. 5 , 693–706 (2009).
Silva, E. C. N., Walters, M. C. & Paulino, G. H. Modeling bamboo as a functionally graded material: lessons for the analysis of affordable materials. J. Mater. Sci. 41 , 6991–7004 (2006).
Korevaar, P. A., Schaefer, C., de Greef, T. F. & Meijer, E. Controlling chemical self-assembly by solvent-dependent dynamics. J. Am. Chem. Soc. 134 , 13482–13491 (2012).
Wang, J. et al. Trace solvent as a predominant factor to tune dipeptide self-assembly. ACS Nano 10 , 2138–2143 (2016).
Hartgerink, J. D., Beniash, E. & Stupp, S. I. Self-assembly and mineralization of peptide-amphiphile nanofibers. Science 294 , 1684–1688 (2001).
Lee, B. K., Yun, Y. & Park, K. PLA micro- and nano-particles. Adv. Drug Deliv. Rev. 107 , 176–191 (2016).
El-Sheikh, S., El-Sherbiny, S., Barhoum, A. & Deng, Y. Effects of cationic surfactant during the precipitation of calcium carbonate nano-particles on their size, morphology, and other characteristics. Colloids Surf. A 422 , 44–49 (2013).
Cheng, J. et al. Formulation of functionalized PLGA–PEG nanoparticles for in vivo targeted drug delivery. Biomaterials 28 , 869–876 (2007).
Moreno‐Alcántar, G. et al. Solvent‐driven supramolecular wrapping of self‐assembled structures. Angew. Chem. Int. Ed. Engl. 133 , 5467–5473 (2021).
Article Google Scholar
Te Brinke, E. et al. Dissipative adaptation in driven self-assembly leading to self-dividing fibrils. Nat. Nanotechnol. 13 , 849–855 (2018).
Albert, J. N. & Epps, T. H. III Self-assembly of block copolymer thin films. Mater. Today 13 , 24–33 (2010).
Knowles, T. P., Oppenheim, T. W., Buell, A. K., Chirgadze, D. Y. & Welland, M. E. Nanostructured films from hierarchical self-assembly of amyloidogenic proteins. Nat. Nanotechnol. 5 , 204–207 (2010).
Rideau, E., Wurm, F. R. & Landfester, K. Self‐assembly of giant unilamellar vesicles by film hydration methodologies. Adv. Biosyst. 3 , 1800324 (2019).
Blanazs, A., Armes, S. P. & Ryan, A. J. Self‐assembled block copolymer aggregates: from micelles to vesicles and their biological applications. Macromol. Rapid Commun. 30 , 267–277 (2009).
Cui, H., Chen, Z., Zhong, S., Wooley, K. L. & Pochan, D. J. Block copolymer assembly via kinetic control. Science 317 , 647–650 (2007).
Rabani, E., Reichman, D. R., Geissler, P. L. & Brus, L. E. Drying-mediated self-assembly of nanoparticles. Nature 426 , 271–274 (2003).
Sorrenti, A., Leira-Iglesias, J., Markvoort, A. J., de Greef, T. F. & Hermans, T. M. Non-equilibrium supramolecular polymerization. Chem. Soc. Rev. 46 , 5476–5490 (2017).
Rivas, C. J. M. et al. Nanoprecipitation process: from encapsulation to drug delivery. Int. J. Pharm. 532 , 66–81 (2017).
Andersen, S. O. Biochemistry of insect cuticle. Annu. Rev. Entomol. 24 , 29–59 (1979).
Gopalan Nair, K. & Dufresne, A. Crab shell chitin whisker reinforced natural rubber nanocomposites. 1. Processing and swelling behavior. Biomacromolecules 4 , 657–665 (2003).
Bogatyreva, N. S., Finkelstein, A. V. & Galzitskaya, O. V. Trend of amino acid composition of proteins of different taxa. J. Bioinform. Comput. Biol. 4 , 597–608 (2006).
Willis, J. H. Structural cuticular proteins from arthropods: annotation, nomenclature, and sequence characteristics in the genomics era. Insect Biochem. Mol. Biol. 40 , 189–204 (2010).
Kajava, A. V. Tandem repeats in proteins: from sequence to structure. J. Struct. Biol. 179 , 279–288 (2012).
Lebouille, J., Stepanyan, R., Slot, J., Stuart, M. C. & Tuinier, R. Nanoprecipitation of polymers in a bad solvent. Colloids Surf. A 460 , 225–235 (2014).
Aryal, S., Hu, C.-M. J. & Zhang, L. Polymer–cisplatin conjugate nanoparticles for acid-responsive drug delivery. ACS Nano 4 , 251–258 (2010).
Hyde, C., Johnson, T. & Sheppard, R. Internal aggregation during solid phase peptide synthesis. Dimethyl sulfoxide as a powerful dissociating solvent. J. Chem. Soc. Chem. Commun. https://doi.org/10.1039/C39920001573 (1992).
Srivastava, K. R., Kumar, A., Goyal, B. & Durani, S. Stereochemistry and solvent role in protein folding: nuclear magnetic resonance and molecular dynamics studies of poly- l and alternating- l , d homopolypeptides in dimethyl sulfoxide. J. Phys. Chem. B 115 , 6700–6708 (2011).
Mendoza-Novelo, B., Mata-Mata, J. L., Vega-González, A., Cauich-Rodríguez, J. V. & Marcos-Fernández, Á. Synthesis and characterization of protected oligourethanes as crosslinkers of collagen-based scaffolds. J. Mater. Chem. B 2 , 2874–2882 (2014).
Gu, L., Jiang, Y. & Hu, J. Scalable spider‐silk‐like supertough fibers using a pseudoprotein polymer. Adv. Mater. 31 , 1904311 (2019).
Annabi, N. et al. Synthesis of highly porous crosslinked elastin hydrogels and their interaction with fibroblasts in vitro. Biomaterials 30 , 4550–4557 (2009).
Nowatzki, P. J. & Tirrell, D. A. Physical properties of artificial extracellular matrix protein films prepared by isocyanate crosslinking. Biomaterials 25 , 1261–1267 (2004).
Ramos, R. et al. Nanocapsules produced by nanoprecipitation of designed suckerin-silk fusion proteins. ACS Macro Lett. 10 , 628–634 (2021).
Zhang, L. et al. Microfluidic synthesis of rigid nanovesicles for hydrophilic reagents delivery. Angew. Chem. Int. Ed. Engl. 127 , 4024–4028 (2015).
Wang, Z., Rutjes, F. P. & van Hest, J. C. pH responsive polymersome Pickering emulsion for simple and efficient Janus polymersome fabrication. Chem. Commun. 50 , 14550–14553 (2014).
Reuvers, A. & Smolders, C. Formation of membranes by means of immersion precipitation: part II. The mechanism of formation of membranes prepared from the system cellulose acetate-acetone-water. J. Membr. Sci. 34 , 67–86 (1987).
Taylor, N. O., Wei, M.-T., Stone, H. A. & Brangwynne, C. P. Quantifying dynamics in phase-separated condensates using fluorescence recovery after photobleaching. Biophys. J. 117 , 1285–1300 (2019).
Ray, S. et al. Mass photometric detection and quantification of nanoscale α-synuclein phase separation. Nat. Chem. 15 , 1306–1316 (2023).
Greenfield, N. J. Analysis of the kinetics of folding of proteins and peptides using circular dichroism. Nat. Protoc. 1 , 2891–2899 (2006).
Vögeli, B., Kazemi, S., Güntert, P. & Riek, R. Spatial elucidation of motion in proteins by ensemble-based structure calculation using exact NOEs. Nat. Struct. Mol. Biol. 19 , 1053–1057 (2012).
Ulijn, R. V. & Smith, A. M. Designing peptide based nanomaterials. Chem. Soc. Rev. 37 , 664–675 (2008).
Nauman, J. V., Campbell, P. G., Lanni, F. & Anderson, J. L. Diffusion of insulin-like growth factor-I and ribonuclease through fibrin gels. Biophys. J. 92 , 4444–4450 (2007).
Cui, H., Webber, M. J. & Stupp, S. I. Self‐assembly of peptide amphiphiles: from molecules to nanostructures to biomaterials. Biopolymers 94 , 1–18 (2010).
Tian, Y., Tirrell, M. V. & LaBelle, J. L. Harnessing the therapeutic potential of biomacromolecules through intracellular delivery of nucleic acids, peptides, and proteins. Adv. Healthc. Mater. 11 , 2270066 (2022).
Tang, L. et al. Investigating the optimal size of anticancer nanomedicine. Proc. Natl Acad. Sci. USA 111 , 15344–15349 (2014).
Midoux, P., Pichon, C., Yaouanc, J. J. & Jaffrès, P. A. Chemical vectors for gene delivery: a current review on polymers, peptides and lipids containing histidine or imidazole as nucleic acids carriers. Br. J. Pharmacol. 157 , 166–178 (2009).
Xiang, Z. et al. Adding an unnatural covalent bond to proteins through proximity-enhanced bioreactivity. Nat. Methods 10 , 885–888 (2013).
Ruoslahti, E. & Pierschbacher, M. D. New perspectives in cell adhesion: RGD and integrins. Science 238 , 491–497 (1987).
Naldini, L. Gene therapy returns to centre stage. Nature 526 , 351–360 (2015).
Pardi, N. et al. Administration of nucleoside-modified mRNA encoding broadly neutralizing antibody protects humanized mice from HIV-1 challenge. Nat. Commun. 8 , 14630 (2017).
Article PubMed PubMed Central Google Scholar
Haopeng, L. et al. Data for: harnessing gradients for self-assembly of peptide-based nanocapsules: a pathway to advanced drug delivery systems. DR-NTU (Data) https://doi.org/10.21979/N9/DJV2BM (2023).
Download references
Acknowledgements
H.L., X.H. and J.Y acknowledge support from the Singapore National Research Fellowship (NRF-NRFF11-2019-0004) and the Singapore Ministry of Education (MOE) Tier 2 Grant (MOE-T2EP30220-0006). T.L. acknowledges support from the National Natural Science Foundation of China (31871959) and the National Key R&D Program of China (2022YFD1700200). Q.Y. acknowledges support from the National Natural Science Foundation of China (32161133010), the National Key R&D Program of China (2022YFD1700200) and the Shenzhen Science and Technology Program (KQTD20180411143628272). X.Q. and H.G. acknowledge support from the Singapore Ministry of Education (MOE) under its Academic Research Fund Tier 1 award no. RG138/20, no. RG135/22 and a start-up grant from Nanyang Technological University, Singapore and A*STAR, Singapore. G.Z and H.G. acknowledge funding support from the Ministry of Education in Singapore under grant MOE-MOET32022-0002. A.M. acknowledges support from the Singapore Ministry of Education (MOE) through an Academic Research (AcRF) Tier 3 grant (Grant No. MOE 2019-T3-1-012). We acknowledge the Facility for Analysis, Characterisation, Testing and Simulation (FACTS) and NTU Institute of Structural Biology (NISB), Nanyang Technological University, Singapore, for use of their HR-TEM, Cryo-TEM and NMR facilities. Molecular dynamics simulations reported were performed on resources provided by the High Performance Computing Centre at Nanyang Technological University, Singapore, and the National Supercomputing Centre, Singapore ( http://www.nscc.sg ).
Author information
These authors contributed equally: Haopeng Li, Xuliang Qian.
Authors and Affiliations
School of Materials Science and Engineering, Nanyang Technological University, Singapore, Singapore
Haopeng Li, Xiao Han, Ali Miserez & Jing Yu
MOE Key Laboratory of Bio-Intelligent Manufacturing, School of Bioengineering, Dalian University of Technology, Dalian, China
Haopeng Li, Huitang Qi, Fenghou Yuan & Tian Liu
School of Mechanical and Aerospace Engineering, Nanyang Technological University, Singapore, Singapore
Xuliang Qian, Guijin Zou & Huajian Gao
School of Biological Sciences, Division of Structural and Computational Biology, Nanyang Technological University, Singapore, Singapore
Harini Mohanram
Institute of High Performance Computing, A*STAR, Singapore, Singapore
Guijin Zou & Huajian Gao
Biological and Biomimetic Material Laboratory (BBML), Center for Sustainable Materials (SusMat), School of Materials Science and Engineering, Nanyang Technological University, Singapore, Singapore
Ali Miserez
State Key Laboratory for Biology of Plant Diseases and Insect Pests, Institute of Plant Protection, Chinese Academy of Agricultural Sciences, Beijing, China
Guangdong Laboratory of Lingnan Modern Agriculture, Genome Analysis Laboratory of the Ministry of Agriculture and Rural Affairs, Agricultural Genomics Institute at Shenzhen, Chinese Academy of Agricultural Sciences, Shenzhen, China
Mechano-X Institute, Applied Mechanics Laboratory, Department of Engineering Mechanics, Tsinghua University, Beijing, China
Huajian Gao
Institute for Digital Molecular Analytics and Science, Nanyang Technological University, Singapore, Singapore
You can also search for this author in PubMed Google Scholar
Contributions
T.L., Q.Y., H.G. and J.Y. designed the study. Q.Y. conceptualized and supported the study. H.L., H.M., X.H., H.Q., F.Y., T.L. and J.Y. conducted the experiments. H.L. performed the experiment and analysis. X.Q. performed the modelling, simulations and analysis. H.L. and X.Q. collected the data. H.L., X.Q., H.M., X.H., H.Q., G.Z., A.M., Q.Y., T.L., H.G. and J.Y. analysed and interpreted the data. The paper was written through contributions of all authors. All authors have given approval of the final version of the paper for submission.
Corresponding authors
Correspondence to Tian Liu , Qing Yang , Huajian Gao or Jing Yu .
Ethics declarations
Competing interests.
J.Y. and H.L. have filed a Singapore provisional patent application number 10202301815S.
Peer review
Peer review information.
Nature Nanotechnology thanks Debasish Haldar and the other, anonymous, reviewer(s) for their contribution to the peer review of this work.
Additional information
Publisher’s note Springer Nature remains neutral with regard to jurisdictional claims in published maps and institutional affiliations.
Supplementary information
Supplementary information.
Supplementary Methods, Tables 1–7 and Figs. 1–45.
Reporting Summary
Source data, source data fig. 1.
Statistical source data.
Source Data Fig. 2
Source data fig. 3, source data fig. 4, source data fig. 5, source data fig. 6, rights and permissions.
Springer Nature or its licensor (e.g. a society or other partner) holds exclusive rights to this article under a publishing agreement with the author(s) or other rightsholder(s); author self-archiving of the accepted manuscript version of this article is solely governed by the terms of such publishing agreement and applicable law.
Reprints and permissions
About this article
Cite this article.
Li, H., Qian, X., Mohanram, H. et al. Self-assembly of peptide nanocapsules by a solvent concentration gradient. Nat. Nanotechnol. (2024). https://doi.org/10.1038/s41565-024-01654-w
Download citation
Received : 19 July 2023
Accepted : 12 March 2024
Published : 26 April 2024
DOI : https://doi.org/10.1038/s41565-024-01654-w
Share this article
Anyone you share the following link with will be able to read this content:
Sorry, a shareable link is not currently available for this article.
Provided by the Springer Nature SharedIt content-sharing initiative
Quick links
- Explore articles by subject
- Guide to authors
- Editorial policies
Sign up for the Nature Briefing: Translational Research newsletter — top stories in biotechnology, drug discovery and pharma.

Member Login
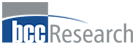
- Med Devices & Surgical
- Cell Biology
- Pharmaceuticals
- Health Maintenance
- Safety and Security
- Engineering
- Advanced Materials
- Semiconductor
- Nanotechnology
- Water-Membrane & Separations
- Fuel Cell & Battery
- Manufacturing
- Environment
- Venture Capital
- Information Technology
- Franchise reports
- BCC Publishing
- Barnes Reports
- Transparency
- Future Markets Insights
- Market Research Studies
- Press Releases
- Association Exclusives
- Partnership
- Innovation Spotlights
- About BCC Research
- Custom Consulting
- Membership Benefits
Peptide Synthesis: Global Markets Projected to Reach $157.5 Billion by 2028, According to BCC Research Analysis
Comprehensive peptide synthesis report: insights into therapeutics and nutraceuticals usage for disease management.
May 02, 2024
Boston: “According to the latest BCC Research study, the demand for Peptide Synthesis: Global Markets is growing from $90.1 billion in 2023 to $157.5 billion by the end of 2028, at a compound annual growth rate (CAGR) of 11.8% from 2023 through 2028.”
The report on peptide synthesis covers everything from the types of peptides to their applications and how they're made. It's like a big picture of the market, including who's doing what, what's selling where, and what's coming up next. It's handy for businesses deciding what to make or invest in and for anyone interested in market trends. The report looks at the market from all angles - from cancer treatments to cosmetics - and breaks it down by regions, like North America and Asia-Pacific. It uses data from 2022 as a starting point and predicts where things are headed by 2028.
Meanwhile, the best-selling drug, Ozempic, will not be available until December 2024 due to high demand and limited production. At the same time, obesity is a silent killer that is increasingly affecting young people, leading to earlier onset of heart disease, and underscoring the urgent need for preventive measures.
To know more about Peptide Synthesis: Global Market Report, click here for details.
Key Drivers of Peptide Synthesis: Global Markets
Developments in Peptide Drug Delivery: Recent advances in drug delivery, such as sustained-release formulations and targeted systems, have improved the efficacy and patient compliance of peptide therapy. New techniques such as nanoparticles and microneedles promise further optimization.
Rising Prevalence of Chronic Diseases: The increase in the number of chronic diseases in the world creates the need for effective treatment. The peptide's specificity, minimal side effects, and pathway-modulating effects make it a promising candidate for the treatment of these diseases, especially as the population ages.
Increase in Biotech Spending: The biotech industry is investing heavily in peptide research and development to drive innovation and market growth. Companies, institutions, and pharmaceutical giants are allocating resources to peptide discovery, synthesis, and clinical trials, accelerating the development of peptide therapies.
Increase in Infectious and Chronic Diseases: Despite advances in treatment, infectious diseases persist, forcing the search for new treatments. Peptides provide a solution with their antimicrobial properties. In addition, peptides play an important role in the treatment of chronic diseases such as cancer and neurodegenerative diseases, demonstrating their versatility in addressing a variety of health problems.
Request a Sample Copy of the Peptide Synthesis: Global Markets Report .
Report Synopsis
Segmentation Analysis:
Synthesis Types: Solid-phase synthesis can efficiently construct peptides on solid supports. Solution-phase synthesis offers flexibility but is less efficient for longer peptides. Mixed-phase synthesis combines both approaches to increase efficiency and flexibility.
Applications: In cosmetics, peptides can improve collagen and improve skin. Nutritional formulations use bioactive peptides to promote health. Peptides are also applicable in agriculture, food, and materials science.
Peptide Therapeutics: In cancer, peptides target specific pathways. In diabetes, they regulate insulin. In neurology, peptides can treat neurodegenerative diseases. They also show promise in cardiology and rare diseases.
Peptide Types: Synthetic peptides are specially designed. Antimicrobial peptides fight infections. Hormonal peptides regulate hormones. Bioactive peptides affect biology. Collagen peptides support skin and joints. Other professional categories serve specific purposes.Top of Form
This report on Peptide Synthesis: Global Market provides a comprehensive insight and analysis that addresses the following key questions:
Some of the Key Market Players Are:
- ABBOTT
- ABBVIE INC.
- AMGEN INC.
- ASTRAZENECA
- BOEHRINGER INGELHEIM INTERNATIONAL GMBH
- BRISTOL MYERS SQUIBB CO.
- B. BRAUN SE
- MERCK & CO. INC.
- MODERNA INC.
- NOVARTIS AG
- NOVO NORDISK A/S
- SANOFI
- TEVA PHARMACEUTICAL INDUSTRIES LTD.
Browse More Related Reports:
Biologic Therapeutic Drugs: Technologies and Global Markets : This report on the biologics market provides a comprehensive analysis of its current state and prospects. The report covers aspects such as competitive dynamics, regulatory environment, and market trends, providing an in-depth understanding of key players and market shares in various product categories such as therapeutic proteins, cell and gene therapies, and vaccines. It also examines market segmentation by application and source, along with a detailed regional analysis. The report uses 2022 as a base year and extends projections to 2028, all in constant dollars.
Global Market for Anti-obesity Drugs : The Anti-Obesity Drugs report provides an overview of the global market, analyzes trends, and forecasts CAGR from 2022 to 2028. Covers pharmaceutical types, distribution channels, and product lines of major companies. Market segmentation includes prescription and over-the-counter drugs distributed through hospitals, retail pharmacies, and online. The regions analyzed include North America, Europe, Asia Pacific, and the rest of the world.
Directly Purchase a copy of the report with BCC Research.
For further information or to make a purchase, please get in touch with [email protected].
Peptide Synthesis: Global Markets( BIO252A ) Publish Date: Apr 2024
Data and analysis extracted from this press release must be accompanied by a statement identifying BCC Research LLC as the source and publisher. For media inquiries, email [email protected] or visit www.bccresearch.com/media to request access to our library of market research.
Join our mailing list for the latest report releases and upcoming event notifications.
- 781-489-7301
- [email protected]
Please select categories of interest:
Search Check All
Optimization of peptide synthesis time and sustainability using novel eco-friendly binary solvent systems with induction heating on an automated peptide synthesizer
Affiliations.
- 1 Interdepartmental Research Unit of Peptide and Protein Chemistry and Biology - Peptlab, MoD&LS Laboratory, University of Florence, Sesto Fiorentino, Italy.
- 2 Department of Chemistry "Ugo Schiff", University of Florence, Sesto Fiorentino, Italy.
- 3 Gyros Protein Technologies Inc., Tucson, Arizona, USA.
- 4 Department of Neurosciences, Psychology, Drug Research and Child Health, Section of Pharmaceutical Sciences and Nutraceutics, University of Florence, Sesto Fiorentino, Italy.
- PMID: 38660732
- DOI: 10.1002/psc.3605
On December 12th, 2023, the European Commission took regulatory action to amend Annex XVII of REACH, imposing restrictions on the use of N,N-dimethylformamide (DMF) within the EU market owing to its high toxicity. Historically, DMF has been widely considered the gold standard for solid-phase peptide synthesis (SPPS). Being urgent to propose alternative solvents, we tested the suitability of non-hazardous neat and mixed solvents. Notably, binary solvent mixtures containing dimethyl sulfoxide as one of the solvent partners demonstrated high efficacy in solubilizing reagents while maintaining the desired swelling characteristics of common resins. A series of binary solvent mixtures were tested in automated SPPS, both at room temperature and high temperature, employing the PurePep® Chorus synthesizer, which enabled controlled induction heating between 25 and 90°C with oscillation mixing. The performances were assessed in challenging peptide sequences, i.e., ACP (65-74), and in longer and aggregating sequences like SARS-CoV-2 RBM (436-507) and β-amyloid (1-42). Furthermore, as part of the proposed sustainable approach to minimize the utilization of hazardous solvents, we coupled the novel PurePep EasyClean catch-and-release purification technology. This work, addressing regulatory compliance, emphasizes the crucial role of green chemistry in advancing safer and more environmentally friendly practices in SPPS.
Keywords: binary mixture solvents; catch and release‐based peptide purification; difficult peptide synthesis; green peptide synthesis; green solvents; induction heating‐assisted peptide synthesis; solid‐phase peptide synthesis.
© 2024 European Peptide Society and John Wiley & Sons Ltd.
Grants and funding
- TT-Pep Well-being Research Incubator Project
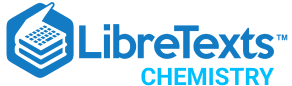
- school Campus Bookshelves
- menu_book Bookshelves
- perm_media Learning Objects
- login Login
- how_to_reg Request Instructor Account
- hub Instructor Commons
Margin Size
- Download Page (PDF)
- Download Full Book (PDF)
- Periodic Table
- Physics Constants
- Scientific Calculator
- Reference & Cite
- Tools expand_more
- Readability
selected template will load here
This action is not available.
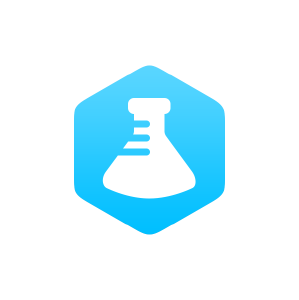
26.7 Peptide Synthesis
- Last updated
- Save as PDF
- Page ID 91062
After completing this section, you should be able to
- describe why it is necessary to protect certain amino and carboxyl groups during the synthesis of a peptide.
- describe, using appropriate equations, how carboxyl groups are protected by ester formation and amino groups are protected by the formation of their tert ‑butoxycarbonyl amide derivatives.
- write a detailed mechanism for the formation of a peptide link between an amino acid with a protected amino group and an amino acid with a protected carboxyl group using dicyclohexylcarbodiimide.
- outline the five steps required in order to form a dipeptide from two given amino acids.
In order to synthesize a peptide from its component amino acids, two obstacles must be overcome. The first of these is statistical in nature, and is illustrated by considering the dipeptide Ala-Gly as a proposed target. If we ignore the chemistry involved, a mixture of equal molar amounts of alanine and glycine would generate four different dipeptides. These are: Ala-Ala, Gly-Gly, Ala-Gly & Gly-Ala . In the case of tripeptides, the number of possible products from these two amino acids rises to eight. Clearly, some kind of selectivity must be exercised if complex mixtures are to be avoided.
The second difficulty arises from the fact that carboxylic acids and 1º or 2º-amines do not form amide bonds on mixing, but will generally react by proton transfer to give salts (the intermolecular equivalent of zwitterion formation ).
From the perspective of an organic chemist, peptide synthesis requires selective acylation of a free amine. To accomplish the desired amide bond formation, we must first deactivate all extraneous amine functions so they do not compete for the acylation reagent. Then we must selectively activate the designated carboxyl function so that it will acylate the one remaining free amine. Fortunately, chemical reactions that permit us to accomplish these selections are well known.
First, the basicity and nucleophilicity of amines are substantially reduced by amide formation . Consequently, the acylation of amino acids by treatment with acyl chlorides or anhydrides at pH > 10, as described earlier , serves to protect their amino groups from further reaction.
Second, acyl halide or anhydride-like activation of a specific carboxyl reactant must occur as a prelude to peptide (amide) bond formation. This is possible, provided competing reactions involving other carboxyl functions that might be present are precluded by preliminary ester formation . Remember, esters are weaker acylating reagents than either anhydrides or acyl halides, as noted earlier .
Finally, dicyclohexylcarbodiimide (DCC) effects the dehydration of a carboxylic acid and amine mixture to the corresponding amide under relatively mild conditions. The structure of this reagent and the mechanism of its action have been described . Its application to peptide synthesis will become apparent in the following discussion.
The strategy for peptide synthesis, as outlined here, should now be apparent. The following example shows a selective synthesis of the dipeptide Ala-Gly.
An important issue remains to be addressed. Since the N-protective group is an amide, removal of this function might require conditions that would also cleave the just formed peptide bond. Furthermore, the harsh conditions often required for amide hydrolysis might cause extensive racemization of the amino acids in the resulting peptide. This problem strikes at the heart of our strategy, so it is important to give careful thought to the design of specific N-protective groups. In particular, three qualities are desired:
- The protective amide should be easy to attach to amino acids.
- The protected amino group should not react under peptide forming conditions.
- The protective amide group should be easy to remove under mild conditions.
A number of protective groups that satisfy these conditions have been devised; and two of the most widely used, carbobenzoxy (Cbz) and t-butoxycarbonyl (BOC or t-BOC), are described here.
The reagents for introducing these N-protective groups are the acyl chlorides or anhydrides shown in the left portion of the above diagram. Reaction with a free amine function of an amino acid occurs rapidly to give the "protected" amino acid derivative shown in the center. This can then be used to form a peptide (amide) bond to a second amino acid. Once the desired peptide bond is created the protective group can be removed under relatively mild non-hydrolytic conditions. Equations showing the protective group removal will be displayed above by are shown above. Cleavage of the reactive benzyl or tert-butyl groups generates a common carbamic acid intermediate (HOCO-NHR) which spontaneously loses carbon dioxide, giving the corresponding amine. If the methyl ester at the C-terminus is left in place, this sequence of reactions may be repeated, using a different N-protected amino acid as the acylating reagent. Removal of the protective groups would then yield a specific tripeptide, determined by the nature of the reactants and order of the reactions.
Contributors and Attributions
Dr. Dietmar Kennepohl FCIC (Professor of Chemistry, Athabasca University )
Prof. Steven Farmer ( Sonoma State University )
William Reusch, Professor Emeritus ( Michigan State U. ), Virtual Textbook of Organic Chemistry
How Peptides Aid in Muscle Building and Recovery
Image Source
The world of fitness is a dynamic one, constantly evolving with new research, techniques, and innovations. Amidst the myriad of supplements and strategies that promise enhanced performance and faster recovery, peptides have emerged as a game-changer. These small chains of amino acids, though seemingly unassuming, have taken the fitness and health industry by storm. Their potential benefits, ranging from muscle growth stimulation to accelerated recovery, have made them a topic of keen interest among athletes, bodybuilders, and casual fitness enthusiasts alike.
But what makes peptides so special? Why have they become the talk of the town in fitness circles? As we embark on this exploration into the realm of peptides, we aim to demystify their role, understand their science, and uncover the reasons behind their rising popularity. Whether you're a seasoned athlete or someone just starting on their fitness journey, this deep dive into peptides promises insights that could revolutionize the way you approach your workouts and recovery.
1. Peptides Defined: More Than Just Protein Fragments
Peptides, in essence, are smaller siblings of proteins. Comprising short chains of amino acids, they play pivotal roles in our body, influencing everything from hormone production to cell signaling. Unlike larger proteins, peptides can quickly penetrate the bloodstream, making them highly efficient. This unique ability has made them a focal point in both therapeutic research and fitness applications.
2. Muscle Growth: The Role of Peptides
Muscle growth isn't just about lifting weights. It's a complex process involving protein synthesis, hormone production, and cellular repair. Peptides, especially growth hormone-releasing peptides, have shown potential in stimulating growth hormone production. This is a game-changer, accelerating cell growth and regeneration. With increased growth hormone levels, athletes and fitness enthusiasts can achieve enhanced muscle growth, pushing their physical boundaries.
GHRP-6 , or Growth Hormone Releasing Peptide-6, is one of the most popular peptides in the fitness community, and for a good reason. This hexapeptide is known for its ability to stimulate the release of growth hormone from the pituitary gland, leading to a host of potential benefits for athletes and fitness enthusiasts.
GHRP-6's primary function is to increase the production of growth hormone, which can lead to increased muscle mass, reduced body fat, and improved recovery times. This makes it a favorite among bodybuilders and those looking to enhance their physical performance. Additionally, GHRP-6 can stimulate appetite, making it useful for individuals looking to increase their caloric intake for muscle building.

3. Speeding Up Recovery with Peptides
Every fitness enthusiast knows that workouts break muscles down, and recovery builds them up. Peptides play a crucial role in this recovery phase. They not only reduce inflammation, ensuring you're ready for your next workout sooner, but also improve sleep quality. As sleep is a prime recovery time, this enhancement ensures muscles repair and grow more efficiently. Additionally, peptides enhance blood flow, ensuring muscles receive all the nutrients they need post-workout.
4. Collagen Peptides: For Joint Health and Beyond
Muscles are just one part of the equation. Joints, often overlooked, play a crucial role in overall fitness. Collagen peptides come into play here. These specific peptides support joint health, ensuring they remain robust and resilient even under intense training regimes. By reducing joint pain and enhancing flexibility, collagen peptides ensure that your skeletal system keeps pace with your muscular growth.
5. Peptides and Fat Metabolism
Lean muscle mass is a goal for many. Peptides can support this by aiding in fat metabolism. Certain peptides promote the breakdown of fat, converting it into energy. This dual benefit not only helps in developing leaner muscle mass but also provides an energy boost. This enhanced energy ensures workouts are more effective, leading to better muscle development.
6. The Connection Between Peptides and Testosterone
Testosterone is synonymous with muscle growth. Certain peptides have shown potential in influencing this vital hormone. By stimulating the pituitary gland, these peptides can indirectly boost testosterone levels. With higher testosterone, muscle development is enhanced, ensuring that every workout yields maximum results.
7. Peptides and Immune System Support
While the primary focus of peptides in the fitness world revolves around muscle growth and recovery, they also play a significant role in bolstering the immune system. A strong immune system is crucial for athletes and fitness enthusiasts, ensuring they remain healthy and can train consistently. Certain peptides have demonstrated the ability to modulate immune responses, helping the body defend against illnesses and infections. This not only ensures uninterrupted training but also aids in overall well-being.
8. The Role of Peptides in Hydration and Skin Health
Fitness isn't just about muscles and strength; it's also about overall health and appearance. Peptides, especially those used in skincare, can enhance skin hydration and elasticity. For athletes, this means faster recovery from minor skin injuries and a youthful appearance. Proper hydration, facilitated by peptides, ensures that the skin remains resilient against external stressors, including the wear and tear of rigorous workouts.
9. Potential Side Effects and Considerations
Every coin has two sides, and peptides are no exception. While they offer numerous benefits, potential side effects cannot be ignored. Overuse can lead to water retention, joint pain, or even hormonal imbalances. It's essential to be informed and cautious. Before incorporating peptides, always consult with a healthcare professional, ensuring that you're making a safe and informed decision.
10. Navigating the World of Peptide Supplements
With the rising popularity of peptides, the market is flooded with various peptide supplements, each promising superior results. But how does one choose the right supplement? It's essential to understand the source, purity, and formulation of these supplements. This section will guide readers through the maze of peptide supplements, ensuring they make informed choices that align with their fitness goals and health considerations.
11. The Future of Peptides in Sports and Bodybuilding
Peptides are not just a passing trend. As research progresses, their potential applications in sports and bodybuilding continue to expand. From enhanced endurance to rapid recovery, the future looks promising. Athletes worldwide might soon consider peptides as essential as their protein shakes, harnessing their benefits for peak performance.
Conclusion:
In conclusion, peptides represent a fascinating intersection of biology and fitness. Their potential in muscle building and recovery is undeniable. As we continue to understand them better, their role in fitness regimens will only grow. For those looking to optimize their workouts, recover faster, and push their limits, peptides might just be the answer.
The post How Peptides Aid in Muscle Building and Recovery appeared first on Things That Make People Go Aww .
- Search Menu
- Advance Articles
- Perspectives
- Knowledgebase and Database Resources
- Nobel Laureates Collection
- China Virtual Outreach Webinar
- Neurogenetics
- Fungal Genetics and Genomics
- Multiparental Populations
- Genomic Prediction
- Plant Genetics and Genomics
- Genetic Models of Rare Diseases
- Genomic Data Analyses In Biobanks
- Why Publish
- Author Guidelines
- Submission Site
- Open Access Options
- Full Data Policy
- Self-Archiving Policy
- About Genetics
- About Genetics Society of America
- Editorial Board
- Early Career Reviewers
- Guidelines for Reviewers
- Advertising & Corporate Services
- Journals on Oxford Academic
- Books on Oxford Academic
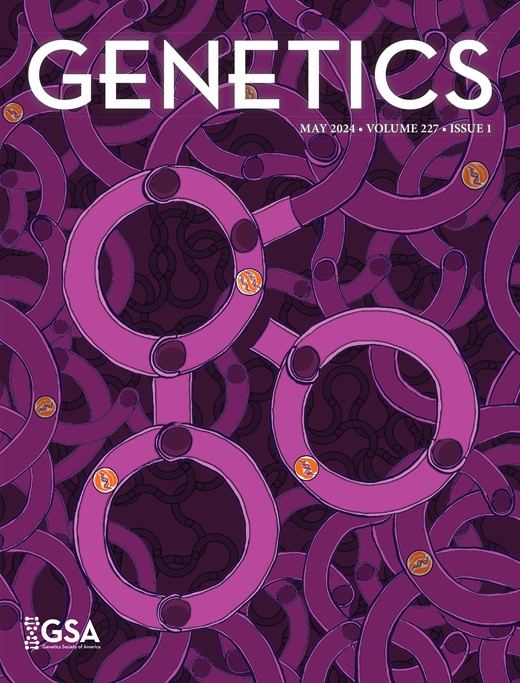
Article Contents
- Introduction
- Materials and methods
- Data availability
- Acknowledgments
- Literature cited
- < Previous
Sex peptide receptor is not required for refractoriness to remating or induction of egg laying in Aedes aegypti

I. Alexandra Amaro and Margot P. Wohl contributed equally.
Conflicts of interest: The author(s) declare no conflict of interest.
- Article contents
- Figures & tables
- Supplementary Data
I Alexandra Amaro, Margot P Wohl, Sylvie Pitcher, Catalina Alfonso-Parra, Frank W Avila, Andrew S Paige, Michelle E H Helinski, Laura B Duvall, Laura C Harrington, Mariana F Wolfner, Conor J McMeniman, Sex peptide receptor is not required for refractoriness to remating or induction of egg laying in Aedes aegypti , Genetics , Volume 227, Issue 1, May 2024, iyae034, https://doi.org/10.1093/genetics/iyae034
- Permissions Icon Permissions
Across diverse insect taxa, the behavior and physiology of females dramatically changes after mating—processes largely triggered by the transfer of seminal proteins from their mates. In the vinegar fly Drosophila melanogaster , the seminal protein sex peptide (SP) decreases the likelihood of female flies remating and causes additional behavioral and physiological changes that promote fertility including increasing egg production. Although SP is only found in the Drosophila genus, its receptor, sex peptide receptor (SPR), is the widely conserved myoinhibitory peptide (MIP) receptor. To test the functional role of SPR in mediating postmating responses in a non- Drosophila dipteran, we generated 2 independent Spr -knockout alleles in the yellow fever mosquito, Aedes aegypti . Although SPR is needed for postmating responses in Drosophila and the cotton bollworm Helicoverpa armigera , Spr mutant Ae. aegypti show completely normal postmating decreases in remating propensity and increases in egg laying. In addition, injection of synthetic SP or accessory gland homogenate from D. melanogaster into virgin female mosquitoes did not elicit these postmating responses. Our results demonstrate that Spr is not required for these canonical postmating responses in Ae. aegypti , indicating that other, as yet unknown, signaling pathways are likely responsible for these behavioral switches in this disease vector.
Email alerts
Citing articles via.
- Recommend to Your Librarian
- Advertising and Corporate Services
- Journals Career Network
Affiliations
- Online ISSN 1943-2631
- Copyright © 2024 Genetics Society of America
- About Oxford Academic
- Publish journals with us
- University press partners
- What we publish
- New features
- Open access
- Institutional account management
- Rights and permissions
- Get help with access
- Accessibility
- Advertising
- Media enquiries
- Oxford University Press
- Oxford Languages
- University of Oxford
Oxford University Press is a department of the University of Oxford. It furthers the University's objective of excellence in research, scholarship, and education by publishing worldwide
- Copyright © 2024 Oxford University Press
- Cookie settings
- Cookie policy
- Privacy policy
- Legal notice
This Feature Is Available To Subscribers Only
Sign In or Create an Account
This PDF is available to Subscribers Only
For full access to this pdf, sign in to an existing account, or purchase an annual subscription.

RSC Advances
Pregnenolone derivatives for the treatment of alzheimer's disease: synthesis, and in vitro inhibition of amyloid β 1–42 peptide aggregation, acetylcholinesterase and carbonic anhydrase-ii †.

* Corresponding authors
a Department of Chemistry, COMSATS University Islamabad, Abbottabad Campus, Abbottabad, Pakistan E-mail: [email protected]
b Department of Pharmacy, University of Malakand, Chakdara, KP, Pakistan
The amyloid state, which is a specific conformation of proteins, offers valuable information about both functional protein structures and the pathological assemblies associated with various diseases. One of the major hallmarks of Alzheimer's disease includes primarily the extracellular build-up of a peptide known as amyloid-β, which has a sequence consisting of 39 to 42 amino acid residues, and the formation of intracellular neurofibrillary tangles mostly consisting of hyperphosphorylated tau protein. Drugs that are expected to reduce Aβ production, prevent Aβ aggregation, and promote Aβ clearance are promising approaches for treating AD. Current work is focused on identifying the compounds that have balanced even mild biological activities against multiple targets instead of finding one-target compound with high potency. We synthesized pregnenolone derivatives and evaluated their potential against inhibition of eeAChE/eqBChE, hCA-II and self-mediated Aβ 1–42 peptide aggregation. Our synthesized derivatives 23 , and 25–27 exhibited concomitant inhibition of all the tested macromolecular targets. All the active compounds were found to be BBB penetrants in the PAMPA assay. Furthermore, these selected compounds were found to be non-neurotoxic in the MTT assay on neuroblastoma SH-SY5Y cells. Docking studies support dual binding site (PAS and CAS) inhibition of AChE which showed Aβ 1–42 aggregation and AChE inhibition. Moreover, docking studies carried out on the 3D crystallographic structure of Aβ 1–42 peptide (PDB ID = 1IYT ) showed significant interactions with amino acid residues Asp 23 and Lys 28, and hydrophobic interactions with the Phe19, Phe20, and Ala 30 effectively impeding the formation of β-sheet structures.
Supplementary files
- Supplementary information PDF (1771K)
Transparent peer review
To support increased transparency, we offer authors the option to publish the peer review history alongside their article.
View this article’s peer review history
Article information

Download Citation
Permissions.

Pregnenolone derivatives for the treatment of Alzheimer's disease: synthesis, and in vitro inhibition of amyloid β 1–42 peptide aggregation, acetylcholinesterase and carbonic anhydrase-II
A. Tahir, B. Mobeen, F. Hussain, A. Sadiq and U. Rashid, RSC Adv. , 2024, 14 , 14742 DOI: 10.1039/D4RA01536C
This article is licensed under a Creative Commons Attribution-NonCommercial 3.0 Unported Licence . You can use material from this article in other publications, without requesting further permission from the RSC, provided that the correct acknowledgement is given and it is not used for commercial purposes.
To request permission to reproduce material from this article in a commercial publication , please go to the Copyright Clearance Center request page .
If you are an author contributing to an RSC publication, you do not need to request permission provided correct acknowledgement is given.
If you are the author of this article, you do not need to request permission to reproduce figures and diagrams provided correct acknowledgement is given. If you want to reproduce the whole article in a third-party commercial publication (excluding your thesis/dissertation for which permission is not required) please go to the Copyright Clearance Center request page .
Read more about how to correctly acknowledge RSC content .
Social activity
Search articles by author, advertisements.
Search Thermo Fisher Scientific
- Order Status
- Quick Order
- Check Order Status
- Aspire Member Program
- Connect: Lab, Data, Apps
- Custom Products & Projects
- Instrument Management
- Home ›
- Life Sciences ›
- Protein Biology ›
- Protein Biology Learning Center ›
- Protein Biology Resource Library ›
- Pierce Protein Methods ›
Peptide Synthesis
- Protein Biology Resource Library
- Pierce Protein Methods
Page contents
- Introduction peptide synthesis
- Applications for synthetic peptides
Process of synthesizing peptides
- Common protecting scheme-specific solvents
- Peptide synthesis strategies
- Peptide purification
View and select products
- Peptide Libraries and Services
- Custom Peptide Synthesis Service
- Protein Isolation and Purification Products
Introduction to peptide synthesis
Peptide synthesis is characterized as the formation of a peptide bond between two amino acids. While there is no definitive definition of a peptide, it usually refers to flexible (little secondary structure) chains of up to 30-50 amino acids.
The ability to form peptide bonds to link amino acids together is over 100 years old, although the first peptides to be synthesized, including oxytocin and insulin, did not occur for another 50-60 years, demonstrating the difficult task of chemically synthesizing chains of amino acids (1). In the last 50 years, advances in protein synthesis chemistry and methods have developed to the point where peptide synthesis today is a common approach in even high-throughput biological research and product and drug development (2).
The benefit of peptide synthesis strategies today is that besides having the ability to make peptides that are found in biological specimens, creativity and imagination can be tapped to generate unique peptides to optimize a desired biological response or other result. This page highlights the important aspects of peptide synthesis, the most common methods of synthesis and purification and the strengths and limitations of the respective strategies.
- Peptide Design
- Peptide Analysis Tool
- Protein Biology Support Centers
Select products
Applications of synthetic peptides.
The invention of peptide synthesis in the fifties and sixties spurred the development of different application areas in which synthetic peptides are now used, including the development of epitope-specific antibodies against pathogenic proteins, the study of protein functions and the identification and characterization of proteins. Furthermore, synthetic peptides are used to study enzyme-substrate interactions within important enzyme classes such as kinases and proteases, which play a crucial role in cell signaling.
In cell biology, receptor binding or the substrate recognition specificity of newly discovered enzymes can often be studied using sets of homologous synthetic peptides. Synthetic peptides can resemble naturally occurring peptides and act as drugs against cancer and other major diseases. Finally, synthetic peptides are used as standards and reagents in mass spectrometry (MS)-based applications. Synthetic peptides play a central role in MS-based discovery, characterization and quantitation of proteins, especially those that serve as early biomarkers for diseases.
- Custom Peptide Synthesis
- KLH-Peptide Conjugation Service
- HeavyPeptide AQUA Custom Synthesis Service
- HeavyPeptide AQUA FasTrack service
- PEPotec SRM Custom Peptide Libraries
- PEPotec Immuno Custom Peptide Libraries
Peptide synthesis most often occurs by coupling the carboxyl group of the incoming amino acid to the N-terminus of the growing peptide chain. This C-to-N synthesis is opposite from protein biosynthesis, during which the N-terminus of the incoming amino acid is linked to the C-terminus of the protein chain (N-to-C). Due to the complex nature of in vitro protein synthesis, the addition of amino acids to the growing peptide chain occurs in a precise, step-wise and cyclic manner. And while the common methods of peptide synthesis have some critical differences, they all follow the same step-wise method to add amino acids one-at-a-time to the growing peptide chain.
Peptide deprotection
Because amino acids have multiple reactive groups, peptide synthesis must be carefully performed to avoid side reactions that can reduce the length and cause branching of the peptide chain. To facilitate peptide formation with minimal side reactions, chemical groups have been developed that bind to the amino acid reactive groups and block, or protect, the functional group from nonspecific reaction.
Purified, individual amino acids used to synthesize peptides are reacted with these protecting groups prior to synthesis, and then specific protecting groups are removed from the newly added amino acid (a step called deprotection) just after coupling to allow the next incoming amino acid to bind to the growing peptide chain in the proper orientation. Once peptide synthesis is completed, all remaining protecting groups are removed from the nascent peptides. Three types of protecting groups are generally used, depending on the method of peptide synthesis, and are described below.
The amino acid N-termini are protected by groups that are termed "temporary" protecting groups, because they are relatively easily removed to allow peptide bond formation. Two common N-terminal protecting groups are tert-butoxycarbonyl ( Boc ) and 9-fluorenylmethoxycarbonyl ( Fmoc ), and each group has distinct characteristics that determine their use. Boc requires a moderately strong acid such as trifluoracetic acid (TFA) to be removed from the newly added amino acid, while Fmoc is a base-labile protecting group that is removed with a mild base such as piperidine.
Boc chemistry was first described in the 1950s and requires acidic conditions for deprotection, while Fmoc, which was not reported for another twenty years, is cleaved under mild, basic conditions (3,4,5,6). Because of the mild deprotection conditions, Fmoc chemistry is more commonly used in commercial settings because of the higher quality and greater yield, while Boc is preferred for complex peptide synthesis or when non-natural peptides or analogs that are base-sensitive are required.
The use of a C-terminal protecting group depends on the type of peptide synthesis used; while liquid-phase peptide synthesis requires protection of the C-terminus of the first amino acid (C-terminal amino acid), solid-phase peptide synthesis does not, because a solid support (resin) acts as the protecting group for the only C-terminal amino acid that requires protection (see Protein Synthesis Strategies).
Amino acid side chains represent a broad range of functional groups and are therefore a site of considerable side chain reactivity during peptide synthesis. Because of this, many different protecting groups are required, although they are usually based on the benzyl (Bzl) or tert-butyl (tBu) group. The specific protecting groups used during the synthesis of a given peptide vary depending on the peptide sequence and the type of N-terminal protection used (see next paragraph). Side chain protecting groups are known as permanent protecting groups, because they can withstand the multiple cycles of chemical treatment during the synthesis phase and are only removed during treatment with strong acids after synthesis is complete.
Because multiple protecting groups are normally used in peptide synthesis, it is evident that these groups must be compatible to allow deprotection of distinct protecting groups while not affecting other protecting groups. Protecting schemes are therefore established to match protecting groups so that deprotection of one protecting group does not affect the binding of the other groups. Because N-terminal deprotection occurs continuously during peptide synthesis, protecting schemes have been established in which the different types of side chain protecting groups (Bzl or tBu) are matched to either Boc or Fmoc, respectively, for optimized deprotection. These protecting schemes also incorporate each of the steps of synthesis and cleavage, as described in the table and in later sections of this page.
Common protecting scheme-specific solvents .
The act of removing protecting groups, especially under acidic conditions, results in the production of cationic species that can alkylate the functional groups on the peptide chain. Therefore, scavengers such as water, anisol or thiol derivatives can be added in excess during the deprotection step to react with any of these free reactive species.
- Amino Acid Physical Properties
Amino acid coupling
Synthetic peptide coupling requires the activation of the C-terminal carboxylic acid on the incoming amino acid using carbodiimides such as dicyclohexylcarbodiimide (DCC) or diisopropylcarbodiimide (DIC). These coupling reagents react with the carboxyl group to form a highly reactive O-acylisourea intermediate that is quickly displaced by nucleophilic attack from the deprotected primary amino group on the N-terminus of the growing peptide chain to form the nascent peptide bond.
Carbodiimides form such a reactive intermediate that racemization of the amino acid can occur. Therefore, reagents that react with the O-acylisourea intermediate are often added, including 1-hydroxybenzotriazole (HOBt), which forms a less-reactive intermediate that reduces the risk of racemization. Additionally, side reactions caused by carbodiimides have led to the examination of other coupling agents, including benzotriazol-1-yl-oxy-tris(dimethylamino)phosphonium hexafluorophosphate (BOP) and 2-(1H-benzotriazol-1-yl)-1,1,3,3-tetramethyluronium hexafluorophosphate (HBTU), which both require activating bases to mediate amino acid coupling.
Peptide cleavage
After successive cycles of amino acid deprotection and coupling, all remaining protecting groups must be removed from the nascent peptide. These groups are cleaved by acidolysis, and the chemical used for cleavage depends on the protection scheme used; strong acids such as hydrogen fluoride (HF), hydrogen bromide (HBr) or trifluoromethane sulfonic acid (TFMSA) are used to cleave Boc and Bzl groups, while a relatively milder acid such as TFA is used to cleave Fmoc and tBut groups. When properly executed, cleavage results in the removal of the N-terminal protecting group of the last amino acid added, the C-terminal protecting group (either chemical or resin) from the first amino acid and any side-chain protecting groups. As with deprotection, scavengers are also included during this step to react with free protecting groups. Because of the importance of cleavage in proper peptide synthesis, this step should be optimized to avoid acid-catalyzed side reactions.
Diagram of peptide cleavage after synthesis. The remaining N-terminal protecting groups, all side-chain protecting groups and the C-terminal protecting group or solid support are removed by strong acid treatment after peptide synthesis is completed.
Liquid-phase peptide synthesis is the classical method that scientists used when first discovering how to generate peptides in vitro and it is still commonly used for large-scale synthesis. This method is slow and labor-intensive, though, because the product has to be manually removed from the reaction solution after each step. Additionally, this approach requires another chemical group to protect the C-terminus of the first amino acid. A benefit of liquid-phase synthesis, though, is that because the product is purified after each step, side reactions are easily detected. Additionally, convergent synthesis can be performed, in which separate peptides are synthesized and then coupled together to create larger peptides.
By far, though, solid-phase peptide synthesis is the most common method of peptide synthesis today. Instead of C-terminal protection with a chemical group, the C-terminus of the first amino acid is coupled to an activated solid support, such as polystyrene or polyacrylamide. This type of approach has a two-fold function: the resin acts as the C-terminal protecting group and provides a rapid method to separate the growing peptide product from the different reaction mixtures during synthesis. As with many different biological manufacturing processes, peptide synthesizers have been developed for automation and high-throughput peptide production.
Although peptide synthesis strategies have been optimized and can be mass-produced, the process to generate peptides is by no means perfect. Events such as incomplete deprotection or reaction with free protecting groups can cause truncated or deletion sequences, isomers or other side products. These events can occur at any step during peptide synthesis, and therefore the longer the peptide sequence, the greater probability that something will negatively affect the synthesis of the target peptide. Thus, peptide yield is inversely correlated with peptide length.
Purification strategies are usually based on a combination of separation methods that exploit the physiochemical characteristics of peptides, including size, charge and hydrophobicity. Purification techniques include:
- Size-exclusion chromatography
- Ion exchange chromatography (IEC)
- Partition chromatography
- High-performance liquid chromatography (HPLC)
Reverse-phase chromatography (RPC) is the most versatile and most widely used method of peptide purification. With traditional methods of HPLC, the stationary phase captures polar, hydrophilic molecules that are then differentially eluted by increasing the concentration of polar solvents in the mobile phase. In RPC, as the name implies, hydrophobic molecules from aqueous solutions are instead captured by the stationary phase using hydrophobic C4, C8 or C18 n-alkyl hydrocarbon ligands, and their retention time is a function of the hydrophobicity of the molecule and that of the mobile phase.
For peptide purification, RPC separates the target peptides from impurities from the synthesis steps, such as isomers, deletion sequences, peptide products from side reactions with free coupling and protecting groups or peptides that have undergone side-chain reactions.
Peptide purity is measured as a percentage of the target peptide to impurities that absorb at the peptide bond absorption wavelength (210-220nm), and varying levels of purity are commercially available based on the application in which the peptides will be used:
- >95% – Quantitative studies such as NMR, receptor-ligand binding studies, ELISA and RIA, monoclonal antibody production, in vivo studies
- >80% – High-throughput screening, non-quantitative blocking in immunohistochemical (IHC) and Western blot analyses, non-quantitative enzyme-substrate studies, antibody affinity purification and plate coating for cell attachment
- >70% – ELISA standards, ELISPOT assays and polyclonal antibody production
- Protein Isolation and Purification Information
- Overview of ELISA
- Antibody Production
- Overview of Immunohistochemistry (IHC)
- Overview of Western Blotting
- Enzyme-linked Immunosorbent Assays (ELISA)
- Immunohistochemistry
- Western Blotting
- Lloyd-Williams P. et al. (1997) Chemical approaches to the synthesis of peptides and proteins. Boca Raton: CRC Press. 278
- Merrifield R. B. (1963) Solid phase peptide synthesis. I. The synthesis of a tetrapeptide. Journal of the American Chemical Society. 85, 2149-54.
- Carpino L. A. (1957) Oxidative reactions of hydrazines. Iv. Elimination of nitrogen from 1, 1-disubstituted-2-arenesulfonhydrazides1-4. Journal of the American Chemical Society. 79, 4427-31.
- McKay F. C. and Albertson N. F. (1957) New amine-masking groups for peptide synthesis. Journal of the American Chemical Society. 79, 4686-90.
- Anderson G. W. and McGregor A. C. (1957) T-butyloxycarbonylamino acids and their use in peptide synthesis. Journal of the American Chemical Society. 79, 6180-3.
- Carpino L. A. and Han G. Y. (1972) 9-fluorenylmethoxycarbonyl amino-protecting group. The Journal of Organic Chemistry. 37, 3404-9.
For Research Use Only. Not for use in diagnostic procedures.

IMAGES
VIDEO
COMMENTS
Peptide synthesis and purification The original sequence peptides were customized from GL Biochem. All those peptides were purified using preparative high-performance liquid chromatography (HPLC).
Comprehensive Peptide Synthesis Report: Insights into Therapeutics and Nutraceuticals Usage for Disease Management May 02, 2024 Boston: “According to the latest BCC Research study, the demand for Peptide Synthesis: Global Markets is growing from $90.1 billion in 2023 to $157.5 billion by the end of 2028, at a compound annual growth rate (CAGR ...
Historically, DMF has been widely considered the gold standard for solid-phase peptide synthesis (SPPS). Being urgent to propose alternative solvents, we tested the suitability of non-hazardous neat and mixed solvents. Notably, binary solvent mixtures containing dimethyl sulfoxide as one of the solvent partners demonstrated high efficacy in ...
A Catalytic Approach for the Synthesis of Peptide–Oligonucleotides Conjugates in Aqueous Solution or On-Column. Marion Gras, Marion Gras. Universite de Montpellier, Chemistry, FRANCE. Search for more papers by this author. ... Peptide–oligonucleotide conjugates (POCs) are covalent architectures composed of a DNA or RNA molecules linked to a ...
Progress in the design and synthesis of nanostructured self-assembling systems has facilitated the realization of numerous nanoscale geometries, including fibers, ribbons, and sheets. A key challenge has been achieving control across multiple length scales and creating macroscopic structures with nanoscale organization. Here, we present a facile extrusion-based fabrication method to produce ...
26.7 Peptide Synthesis. Page ID. Objectives. After completing this section, you should be able to. describe why it is necessary to protect certain amino and carboxyl groups during the synthesis of a peptide.
2. Muscle Growth: The Role of Peptides. Muscle growth isn't just about lifting weights. It's a complex process involving protein synthesis, hormone production, and cellular repair.
Although SP is only found in the Drosophila genus, its receptor, sex peptide receptor (SPR), is the widely conserved myoinhibitory peptide (MIP) receptor. To test the functional role of SPR in mediating postmating responses in a non- Drosophila dipteran, we generated 2 independent Spr -knockout alleles in the yellow fever mosquito, Aedes aegypti .
Pregnenolone derivatives for the treatment of Alzheimer's disease: synthesis, ... One of the major hallmarks of Alzheimer's disease includes primarily the extracellular build-up of a peptide known as amyloid-β, which has a sequence consisting of 39 to 42 amino acid residues, and the formation of intracellular neurofibrillary tangles mostly ...
Get a detailed intro on peptide synthesis and how they are used to prepare epitope-specific antibodies, map antibody epitopes, and enzyme binding sites.